In recent years, research has focused on strategies for decreasing disease relapse rate. Methods manipulating the graft immune activity towards GVL with minimizing GVHD represent a new exciting direction which could promote the next breakthrough in allogeneic HSCT. Graft-versus-leukemia is mainly attributed to the immune response of donor T lymphocytes against residual or relapsed leukemic cells, as well as to the immune response of natural killer (NK) cells and B lymphocytes.49 Among the leading strategies that have already reached the clinical phase of investigation are graft manipulations in the setting of haploidentical HSCT, vaccinations against tumor-associated antigens (TAA), monoclonal antibodies, and targeted adoptive cellular immunotherapy.
Regulatory T Cell Infusion
Haploidentical HSCT allows for immediate donor availability for 95% of patients. However, its use has been limited by a significant rate of non-engraftment and infections.
32 One strategy to overcome these issues is to engineer the graft and replete it with regulatory and effector T cells in a specific ratio. Human natural regulatory T cells (Tregs) derive from the thymus and express high levels of CD25 (interleukin 2 receptor alpha) and intracellular Foxp3 (forkhead box P3) which is a master switch transcription factor.
50,51 Tregs suppress other active conventional T cell (Tcon) populations allowing for immune homeostasis and therefore have a role in preventing autoimmunity and limiting inflammatory disease.
52 Tregs were shown to be capable of inducing immune tolerance in animal models of bone marrow transplantation, thus preventing GVHD without hampering GVL.
53–55 To date, several phase I–II clinical trials have been conducted. One study was performed in the setting of double UCB transplantation, in which third-party UCB Tregs were
ex vivo expanded and infused. The procedure was shown to be safe with no increased rates of GVHD, infections, or disease relapse.
56 In another study pre-emptive Treg DLI was performed after stopping immunosuppression, in order to prevent relapse after allogeneic HSCT in high-risk patients.
57 In one study, Treg DLI from a donor was given as treatment for active chronic GVHD after allogeneic HSCT.
58 In two other studies in the haploidentical HSCT setting, Tregs were infused a few days prior to the transfusion of a TCD stem cell graft combined with Tcon infusion. The Treg and Tcon ratio was 2:1, and the purpose of the study was to use the Tregs as the sole GVHD prophylaxis strategy and as a way to improve immune reconstitution without increasing disease relapse rate.
59,60 While GVHD and relapse rates in these studies were lower compared to historical controls, non-relapse mortality is still unsatisfactory.
Graft Depletion of αβ CD19 T Cells
The αβ T cell receptor (TCR)-positive T cells constitute 95% of the T cell population. These lymphocyte subsets are responsible for the occurrence of GVHD.
61 γδ T cells (also termed “innate-like” T cells or “transitional” T cells) belong to the adoptive arm of the immune system.
62 They are capable of recognizing their targets in a major histocompatibility complex-independent manner; therefore, they are unlikely to elicit GVHD. Higher levels of γδ TCR-positive T cells were found to correlate with better leukemia-free survival in haploidentical HSCT recipients.
63 This finding led to two phase I–II clinical trials in the setting of haploidentical HSCT. Grafts were depleted from αβ T cells, allowing for the transplantation of γδ lymphocyte retained grafts. No other prophylactic treatment was given for GVHD prevention.
64,65 This method allowed a sustained engraftment, rapid immune reconstitution, and low incidence of both acute and chronic GVHD with a comparable non-relapse mortality rate to that of MUD. Regarding disease relapse the follow-up is still too short.
Natural Killer Cell Adoptive Immunotherapy
In TCD haploidentical HSCT it was shown that mature fully functioning NK cells derived from differentiation of hematopoietic stem cells emerge in the peripheral blood of the recipient only several weeks after the allograft, while in the early post-transplant period immature poorly functioning NK cells predominate.
66 Therefore patients receiving allografts from haploidentical NK alloreactive relative donors cannot benefit from NK-mediated GVL effect in the early post-transplant period. This led to a phase II trial of pre-emptive infusion of donor NK cells (day 4, day 30, and day 100 post-transplant) with an aim of preventing graft failure and adding to GVL effect without increasing GVHD.
66 Compared to historical controls there was no advantage in preventing graft failure or disease relapse. However, this treatment is safe and feasible and should be further explored regarding NK cell dose, timing, and need for activation of the NK cells prior to their transfusion.
Anti-Tumor Vaccination
Autologous primed T cells targeted against specific tumor-associated antigens or against the whole tumor cell have been developed and tested in acute myeloid leukemia (Wilms tumor-associated antigen 1, WT1), chronic myeloid leukemia (BCR-ABL peptide epitopes), and multiple myeloma (dendritic cell/myeloma fusion cells). Many trials have tested augmenting the patient immune response against the tumor in the autologous setting.
68,69 In two trials, autologous inactivated leukemia cells were transfused to patients following allogeneic stem cell transplantation to induce
in vivo immunity. The procedure was proved to be safe, immunogenic, and associated with biological activity despite the use of immunosuppression for GVHD prophylaxis.
70,71 However, this treatment may have significant advantage in the setting of allogeneic HSCT allowing for the transfer of targeted adoptive immunotherapy specific against the tumor with no risk for GVHD. By this approach vaccinating the donor before lymphocyte collection has the advantage of vaccine-primed lymphocytes collected from a healthy donor with healthy immune system, rather than from patients tolerant to their own tumor antigens with reduced immunity from prior chemotherapy. It has been tested in a small number of patients with multiple myeloma, where the donors were immunized with the recipient myeloma paraprotein. In one case the donor was immunized prior to DLI collection to treat relapsed disease after the HSCT.
72 In the other cases the donor was immunized before stem cell collection, and the recipient showed immunogenic response with long-term remission.
73,74 Further trials are required to find immunogenic targets and immunization strategies in the allogeneic HSCT setting. The main limitation is due to the need to perform the vaccination procedures on a healthy donor. Priming and expanding donor T cells
ex vivo against patient TAA will resolve the issue of vaccinating the patient himself; however, this will require very high costs.
Monoclonal Antibodies
Monoclonal antibodies (mAb) are in routine use in the treatment of hematologic malignancies. Rituximab, an anti-CD20 antibody, is the standard treatment protocol for B cell lymphomas. Gemtuzumab ozogamicin, a humanized anti-CD33 mAb conjugated to calicheamicin-derivate toxin, has been used in AML and been shown when incorporated in low dose with chemotherapy to result in improved overall survival.
75 Brentuximab vedotin, an anti-CD30 mAb linked to monomethyl auristatin E, a microtubule-disrupting agent, has revolutionized treatment in Hodgkin lymphoma and CD30-positive peripheral T cell lymphoma.
76 Now in preclinical and clinical trials are the bispecific T cell engager antibodies (BiTEs). These antibodies bind target tumor cells and at the same time bind and harness polyclonal cytotoxic T cells to cause highly efficient lysis of targeted tumor cells. Blinatumumab, an anti-CD3/CD19 BiTE mAb, has been tested in relapsed and refractory B cell acute lymphoblastic leukemia patients.
77 Other CD3/CD33 BiTEs have shown excellent results in preclinical trials for AML.
78,79 New alternative antigenic targets in AML are being investigated.
80 The role of these mAb in the setting of allogeneic HSCT has yet to be determined.
Chimeric Antigen Receptors
Chimeric antigen receptors (CARs) are recombinant receptors that provide both antigen-binding and T cell-activating functions (
Figure 2). The engineering of CARs into T cells requires that T cells be cultured to allow for gene transduction and stable clonal expansion. Any cell surface molecule can be targeted through a CAR. Current CARs are limited to recognizing only cell surface antigens (T cell receptors recognize both cell surface and intra-cellular proteins). However, CARs do not require antigen processing and presentation by HLA. Therefore CARs recognize antigen on any HLA background, in contrast to T cell receptors (TCR), which need to be matched to the patient’s haplotype. Furthermore, CARs can target tumor cells that down-regulate HLA expression or use proteasomal antigen processing, two mechanisms that contribute to tumor escape from TCR-mediated immunity.
81 The most investigated target to date is CD19 found on B cell lymphocyte and on malignancies arising from it (B cell non-Hodgkin lymphoma, chronic lymphocytic leukemia, B cell acute lymphoblastic leukemia). Several phase I–II studies have been conducted in patients with very refractory disease, with some of the studies showing promising results.
82–85 Novel targets are being investigated in preclinical setting like the promising CARs against CD123, which is found on acute myeloid leukemia cells.
86,87 Chimeric antigen receptor T cell adoptive therapy seems to have a great potential, and its best effect might be in the allogeneic HSCT setting.
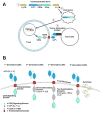 | Figure 2. The Chimeric Antigen Receptor (CAR). |
Selective Allo-Depleted T Cells
T cell depletion abrogates GVHD completely with higher rates of non-engraftment, infections, and disease relapse. Host alloreactive donor lymphocytes are responsible for GVHD. Theoretically selective elimination of host alloreactive lymphocytes from the graft will result in significant reduction in GVHD rate without effecting GVL and immune reconstitution. Selective allodepletion methods rely on the co-culturing of irradiated host peripheral blood mononuclear cells with donor T cells. Alloreactive T cells are activated, identified, and removed either with monoclonal antibodies coupled to magnetic beads or photodepletion procedure (
Figure 3). Peripheral blood stem cells are collected from the donor with leukopheresis. The graft is then T cell-depleted by positive stem cell selection. The unabsorbed T cells are used for the selective depletion. On the transplantation day the patient receives the graft and the selective allodepletion T cells.
88 Few clinical trials have been carried out to prove the concept.
89,90 However, the procedure is laborious and expensive, and necessitates a “good manufacturing practice” facility.
Antiviral Cytotoxic Cell Lines
Viral infections are a significant cause of morbidity and mortality in transplantation, especially in pediatric patients, and particularly in TCD haploidentical transplantation and in UCB transplantation. Effective therapies are limited in refractory infections and often associated with significant side effects. Adoptive transfer of virus-reactive T cells offers a means of reconstituting antiviral immunity, and this approach has been successfully used to prevent and treat cytomegalovirus, Epstein–Barr virus, and adenovirus infections. Adoptive antiviral cytotoxic T cell lines have been used effectively for more than a decade. However, this therapy necessitates a “good manufacturing practice” facility.
91