| |
|
RMMJ
Rambam Maimonides Medical Journal
Rambam Health Care Campus 2017 April; 8(2): e0028.
ISSN: 2076-9172 Published online 2017 April 28. doi: 10.5041/RMMJ.10305
Special Issue on Gynecology, Fertility, and Obstetrics
Guest Editors: Lior Lowenstein, M.D., M.S., M.H.A., Shahar Kol, M.D., and Zeev Weiner, M.D.
Maternal Inflammation, Fetal Brain Implications and Suggested Neuroprotection: A Summary of 10 Years of Research in Animal Models Yuval Ginsberg, M.D.,1* Nizar Khatib, M.D.,1 Zeev Weiner, M.D.,1,2 and Ron Beloosesky, M.D.1,2 1Department of Obstetrics and Gynecology, Rambam Health Care Campus, Haifa, Israel 2The Ruth & Bruce Rappaport Faculty of Medicine, Technion–Israel Institute of Technology, Haifa, Israel
|
A growing body of evidence implies that maternal inflammation during pregnancy is associated with increased risk of neurodevelopmental disorders in the offspring. The pathophysiological mechanisms by which maternal inflammation evokes fetal brain injury and contributes to long-term adverse neurological outcomes are not completely understood. In this review, we summarize 10 years of our research experience on maternal inflammation and the implications upon the fetal/offspring brain. We review our findings regarding the underlying mechanisms that connects maternal inflammation and fetal brain injuries (e.g. cytokines, oxidative stress); we discuss our imaging, pathological and behavioral test results which support brain damage following maternal inflammation; and finally we describe some of the therapeutic strategies which might prevent the damage.
Keywords: Fetal brain injury, magnesium sulfate, maternal inflammation, N-acetyl cysteine neuroprotection |
For many years, intrapartum hypoxia/asphyxia was considered the main etiology for cerebral palsy and fetal brain injuries. In reality, new epidemiological studies have revealed that asphyxia accounts for only 20% of the cases of cerebral palsy, and 70% of the cases arise even before the onset of labor.1 In recent years, a plethora of both clinical and epidemiological studies has established a correlation between an “adverse” intrauterine environment and abnormal development of the fetal brain. While the consequences of chorioamnionitis for both cerebral palsy and adverse neurologic injuries are clear,2–6 maternal inflammation that occurs during critical periods of fetal development has also been identified as a significant risk factor for some neuropsychiatric and neurobehavioral disorders, including schizophrenia, autism and cognitive delay.7–13 However, the mechanism by which maternal infection and/or inflammation of the uterine cavity can evoke fetal brain injury and contribute to long-term adverse neurological outcomes remains unclear. This is a summary of our 10-year14–23 research using a rat model of maternal inflammation for a better understanding of the mechanisms by which maternal inflammation/infection may attenuate normal fetal brain development, and optional neuroprotective interventions. |
Systemic Versus Local Inflammation To understand the mechanisms associated with human fetal brain injury, a model with similar developmental milestones and brain structure proportions should be found. The insult should be delivered at an equivalent stage of intrauterine brain development for an identical period of vulnerability. Measurement of the outcomes of the insult should be feasible by acceptable methods. Clearly, no animal model can fully mimic the development of the human brain, and each species has its own characteristics.
Several types of animal models have been suggested for elucidating the mechanisms that link prenatal inflammation and adverse fetal brain development.20,24–28 Each animal model has its own clinical advantages. Rodent models have been used widely to investigate the influence of local insult on preterm delivery and fetal brain development.25,27–31 Most of these studies used local delivery of an insult (uterine injection of lipopolysaccharide [LPS], for example) to mimic a well-documented mechanism of ascending intrauterine infection, which might cause preterm birth or chorioamnionitis. Systemic models that mimic clinical scenarios of maternal infection/inflammation during gestation have received less attention.
Timing of the Insult The development and maturation of the human brain is a complex and continuous process. Primary neurulation occurs during weeks 3–4, neuronal proliferation during months 3–4, migration during months 3–5, and myelination begins during the second trimester and continues into adulthood. Hence, as mentioned above, the timing of an insult is crucial to compare and evaluate the neurodevelopmental response of offspring. While early insult is associated with structural brain abnormalities such as neural tube defects (primary neurulation), later insults may disrupt the migration process of post-mitotic neurons and lead to aberrant cortical development. 28 Late-gestation insults have been found to associate more with cognitive, behavioral, and psychiatric disorders, such as schizophrenia, autism, and obsessive compulsive disorders. 27,32,33 |
Both epidemiological and experimental studies in animals have demonstrated that maternal infection can damage the developing brain.34–37 It was suggested that infections activate inflammatory pathways, causing the release of various proinflammatory biomarkers, such as cytokines, interleukins, and other molecules. Cytokines are intracellular mediators that are crucial to counter infections. While proinflammatory cytokines mobilize immune system cells to proliferate, to produce more cytokines, and to encourage the inflammatory cascade, anti-inflammatory cytokines act to depress and control the inflammatory response. Since cytokines are 50 kDa, the potential transfer of cytokines between a mother, a fetus, and amniotic fluid might occur.
Proinflammatory cytokines such as tumor necrosis factor-alpha (TNF-α), interleukin (IL) 1β, and IL-6 from astrocytes and microglia may directly damage oligodendrocytes and neurons. For example, the injection of IL-1β leads to neuronal death and delayed myelination in neonatal rats.38 Tumor necrosis factor-α induces cell death in mature oligodendrocytes and apoptosis in developing oligodendrocytes.39,40 Exposure to TNF-α appears to be associated with reduced myelination and diffuse white matter damage in fetal rodents24 and in preterm infants.41 The production of free radicals following the initial neural injury exacerbates collateral damage for neurogenesis and neurodifferentiation (Figure 1).28,37,42 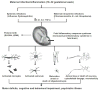 | Figure 1 Suggested Mechanism of Fetal Brain Injury Following Maternal Inflammation. |
|
MEASURING THE CYTOKINE RESPONSE
In light of the potential contribution of cytokines to fetal neurological injury, we first sought to evaluate fetal inflammatory responses to maternal inflammation. One common method of mimicking inflammation associated with infection in animal models is through the exposure of modified proteins. Lipopolysaccharide is a Gram-negative bacterial component that mimics bacterial infections, and LPS exposure was found to induce the production of IL-6, IL-1β, and TNF-α. We used intraperitoneal (i.p.) injections of LPS to induce maternal systemic inflammation in pregnant rats.16–18,21,28,43
In response to maternal i.p. injections of LPS, TNF-α was the first cytokine to peak; this was followed by a quick return to baseline in both maternal circulation and amniotic fluid. Interleukin-1β and IL-6 had delayed responses. Assessment of changes in the mRNA levels of these proinflammatory cytokines revealed that LPS induced increases in TNF-α, IL-6, and IL-10, both in the chorio-amnion and in the placenta. Similar results were demonstrated in the fetal brain (Figure 2). 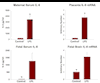 | Figure 2 Maternal Serum, Placenta, Fetal Serum, and Fetal Brain Levels of IL-6 Following Maternal Inflammation. |
Our results imply that acute maternal inflammation can affect the proinflammatory status of the amniotic fluid, and the chorio-amnion membranes, fetal serum, and brain. All of them are associated with fetal brain injury. Furthermore, we recognize that time does matter. In early gestation, the fetal anti-inflammatory response is decreased due to the immature immune system. Therefore, the earlier maternal inflammation occurs, the worse the prognosis. |
MEASURING THE OXIDATIVE STRESS RESPONSE
Increased maternal oxidative stress in pregnancy is associated with an increased risk of poor pregnancy outcome. Several studies have shown that markers of oxidative stress are increased during maternal inflammation. Following maternal LPS injection, elevated oxidative stress has been observed in rat fetus brains several hours and days after delivery.14,22,44,45 As previously mentioned, proinflammatory cytokines induce oxidative stress mediators. In a “vicious cycle” mechanism, oxidative stress rises, increasing cytokine induction. Using our rat model,14,22 we demonstrated that maternal inflammation induces oxidative stress in the maternal serum and amniotic fluid, and increases the basal oxidative state in neonates.
In another study,15 we demonstrated that LPS-induced maternal inflammation at 16 days of gestational (E16) and 18 days of gestation (E18) significantly increased fetal brain phosphor-neuronal nitric oxide synthase, nuclear factor kappa-light-chain-enhancer of activated B cells (NF-κB) p65, and chemokine (C-C motif) ligand 2 protein levels. |
Our group pioneered the use of magnetic resonance imaging (MRI) to demonstrate the long-term consequences of maternal inflammation on fetal brain development. By using advanced MRI methods,17 such as T2 relaxation time, diffusion tensor imaging (DTI), and apparent diffusion coefficient (ADC), we demonstrated that offspring of LPS-treated dams, 25 days postnatal, exhibited significant changes in both white and gray matter (e.g. hypothalamus, motor cortex, corpus callosum, thalamus, hippocampus), consistent with diffuse cerebral injury. |
CLINICAL ASPECTS OF BRAIN INJURY—LEARNING AND MEMORY ABILITIES
As evident by MRI, offspring of LPS-treated dams exhibited white and gray matter injury. Many of these areas were in regions known to be involved in learning and memory, including the dorsal striatum, medial septal nucleus, entorhinal cortex, and molecular dentate gyrus.17,18 To translate laboratory and imaging data into clinical practice, we decided to examine learning and memory abilities of neonates following maternal inflammation. We used the two-way shuttle avoidance box test to evaluate learning and memory abilities. Our data demonstrated that maternal inflammation significantly attenuates learning abilities of 3-month-old offspring (Figure 3).23 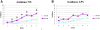 | Figure 3 Neonate Learning Deterioration Following Maternal Inflammation. |
|
THERAPEUTIC STRATEGIES OF NEUROPROTECTION
Since maternal infection/inflammation increases offspring brain cytokines and free radicals, both anti-inflammatory substances and free radical scavengers might have therapeutic properties that prevent fetal adverse neurologic outcomes.
Magnesium Sulfate During the last decade, several prospective studies in pregnant patients have demonstrated the neuroprotective effect of magnesium sulfate (MG) in preventing preterm white matter brain injury. 46–50 Following the Cochrane meta-analysis review, 51 MG became “the drug of choice” for preventing brain injuries and cerebral palsy in “at-risk” fetuses. In light of the importance of preventing newborn brain injury, and although the neuroprotective mechanism of MG has not been elucidated, we sought to investigate the use of MG in preventing short- and long-term fetal brain consequences of LPS-induced maternal inflammation.
Oxidative Stress and Inflammatory Modulators Following Magnesium Sulfate Administration. Recently we reported15 that MG treatment of LPS dams significantly decreased fetal brain phospho-nNOS, NF-κB, and CCL2 protein levels that presented subsequent to maternal administration of LPS. This study reports, for the first time, that acute maternal inflammation can induce an inflammatory response in the fetal brain through direct activation of fetal brain NF-κB and phospho-nNOS, and that maternal MG attenuates this response through N-methyl-D-aspartate receptor (NMDA-R) (Figure 4). 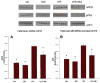 | Figure 4 MgSO4 Decreases Levels of Fetal Brain nNOS and NF-κB Following Maternal Inflammation. |
Fetal Brain Imaging Following Magnesium Sulfate Administration. Maternal LPS at E18 induces brain injury in offspring at 25 days of age, evident by MRI.18 The injury was demonstrated in both gray and white matter areas. Magnesium sulfate treatment administered for 2 hours prior to and following i.p. LPS prevented offspring brain injury as demonstrated by MRI (Figure 5; Table 1). 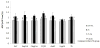 | Figure 5 MgSO4 Decreases Fetal Brain Injury Following Maternal Inflammation. |
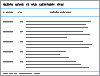 | Table 1 Affected Fetal Brain Region Function. |
Learning and Memory Abilities. As described before,23 we used the two-way shuttle avoidance test to investigate neurobehavioral outcomes associated with maternal inflammation and the impact of neuroprotection with MG. Our results demonstrated a clear decline in learning abilities of the offspring of LPS-treated dams and a return to normal values following treatment with MG. Interestingly, at age 3 months the abilities of the LPS-MG group exceeded those of all the other groups, including the control-saline and the MG-only group (Figure 6). The reason for this unexpected result is unclear. 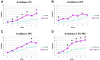 | Figure 6 MgSO4 Improves Neonate Learning and Memory Abilities Following Maternal Inflammation. |
Our results support the notion that MG protection is not limited to the white matter, but that it may protect the gray matter and cognitive functions of the offspring as well.
N-Acetyl Cysteine. N-acetyl cysteine (NAC) is an antioxidant that scavenges free oxygen radicals. In addition, NAC can act indirectly as a stimulant of synthesis of the anti-stressogenic agent glutathione.52,53 The effectiveness of NAC as a neuroprotective has been already well established. In a hypoxia-ischemia rat model, Wang et al.54 demonstrated that treatment with NAC significantly decreased brain injury in LPS-sensitized pups. As previously mentioned, since the inflammatory reaction might cause accumulation of both cytokines and oxygen free radicals, we thought to use NAC to reduce the inflammatory reaction and to narrow the risk of fetal brain injury.
Cytokines. Following LPS-induced maternal inflammation, NAC administration significantly reduced pro-inflammatory cytokine expression in the maternal circulation, amniotic fluid, fetal blood, and most importantly in the fetal brain (Figure 7).14,16,17,20 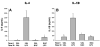 | Figure 7 NAC Decreases Fetal Plasma IL-6 and IL-1β Levels Following Maternal Inflammation. |
Fetal Brain Imaging Following NAC Administration. Maternal NAC administration following the i.p. LPS-induced maternal inflammation prevented any visible damage to the offspring neonatal brains17 (Figure 8; Table 1). 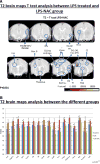 | Figure 8 NAC Decreases Fetal Injury as Demonstrated by MRI Following Maternal Inflammation. |
Our findings suggest that NAC might attenuate the short- and long-term sequelae associated with a fetal brain inflammatory response in pregnant women affected by severe inflammation. Although these were encouraging results concerning the influence of NAC upon both fetal and maternal immune responses, further studies regarding this are still needed. |
Maternal inflammation during pregnancy is associated with an increased risk of neurodevelopmental disorders in the offspring. Activation and up-regulation of inflammatory cytokines and oxidative stress, both systemically and in the fetal brain, are thought to play a key role in altered brain development and may contribute to poor neurodevelopmental outcomes subsequent to maternal inflammation. Possible therapeutic intervention may include antioxidative and anti-inflammatory therapies aimed to reduce the severity and extent of the injury.
In order to develop realistic therapeutic options, future research is needed to clarify the mechanisms linking maternal inflammation, fetal brain development, and neurological and behavioral deficits. |
ADC |
apparent diffusion coefficient |
DTI |
diffusion tensor imaging |
IL |
interleukin |
IL-1β |
interleukin 1β |
i.p. |
intraperitoneal |
LPS |
lipopolysaccharide |
MRI |
magnetic resonance imaging |
MG |
magnesium sulfate |
NAC |
N-acetyl cysteine |
NOS |
nitric oxide synthase |
NS |
normal saline |
NF-κB |
nuclear factor kappa-light-chain-enhancer of activated B cells |
NMDA-R |
N-methyl-D-aspartate receptor |
TNF-α |
tumor necrosis factor-alpha |
|
|
2. Pickler R, Brown L, McGrath J, et al. Integrated review of cytokines in maternal, cord, and newborn blood: Part II -- Associations with early infection and increased risk of neurologic damage in preterm infants. Biol Res Nurs. 2010;11:377–86. https://doi.org/10.1177/1099800409344619. 4. Pinelli J, Zwaigenbaum L. Chorioamnionitis, gestational age, male sex, birth weight, and illness severity predicted positive autism screening scores in very-low-birth-weight preterm infants. Evid Based Nurs. 2008;11:122. https://doi.org/10.1136/ebn.11.4.122. 7. Jones KL, Croen LA, Yoshida CK, et al. Autism with intellectual disability is associated with increased levels of maternal cytokines and chemokines during gestation. Mol Psychiatry. 2017;22:273–9. https://doi.org/10.1038/mp.2016.77. 8. Goines PE, Croen LA, Braunschweig D, et al. Increased midgestational IFN-γ, IL-4 and IL-5 in women bearing a child with autism: a case-control study. Mol Autism. 2011;2:13. https://doi.org/10.1186/2040-2392-2-13. 9. Khandaker GM, Zimbron J, Lewis G, Jones PB. Prenatal maternal infection, neurodevelopment and adult schizophrenia: a systematic review of population-based studies. Psychol Med. 2013;43:239–57. https://doi.org/10.1017/S0033291712000736. 10. Yamashita Y, Fujimoto C, Nakajima E, Isagai T, Matsuishi T. Possible association between congenital cytomegalovirus infection and autistic disorder. J Autism Dev Disord. 2003;33:455–9. https://doi.org/10.1023/A:1025023131029. 11. Sorensen HJ, Mortensen EL, Reinisch JM, Mednick SA. Association between prenatal exposure to bacterial infection and risk of schizophrenia. Schizophr Bull. 2009;35:631–7. https://doi.org/10.1093/schbul/sbn121. 13. Urakubo A, Jarskog LF, Lieberman JA, Gilmore JH. Prenatal exposure to maternal infection alters cytokine expression in the placenta, amniotic fluid, and fetal brain. Schizophr Res. 2001;47:27–36. https://doi.org/10.1016/S0920-9964(00)00032-3. 14. Awad N, Khatib N, Ginsberg Y, et al. N-acetyl-cysteine (NAC) attenuates LPS-induced maternal and amniotic fluid oxidative stress and inflammatory responses in the preterm gestation. Am J Obstet Gynecol. 2011;204:450.e15–20. https://doi.org/10.1016/j.ajog.2011.01.030. 15. Beloosesky R, Khatib N, Ginsberg Y, et al. Maternal magnesium sulfate fetal neuroprotective effects to the fetus: inhibition of neuronal nitric oxide synthase and nuclear factor kappa-light-chain-enhancer of activated B cells activation inthom. Am J Obstet Gynecol. 2016;215:382.e1–6. https://doi.org/10.1016/j.ajog.2016.03.032. 16. Beloosesky R, Gayle DA, Ross MG. Maternal N-acetylcysteine suppresses fetal inflammatory cytokine responses to maternal lipopolysaccharide. Am J Obstet Gynecol. 2006;195:1053–7. https://doi.org/10.1016/j.ajog.2006.06.081. 17. Beloosesky R, Ginsberg Y, Khatib N, et al. Prophylactic maternal N-acetylcysteine in rats prevents maternal inflammation-induced offspring cerebral injury shown on magnetic resonance imaging. Am J Obstet Gynecol. 2013;208:213.e1–213.e6. https://doi.org/10.1016/j.ajog.2013.01.023. 18. Beloosesky R, Ginsberg Y, Weiner Z, et al. Magnesium sulphate (Mg) prevents maternal inflammation-induced offspring cerebral injury evident by magnetic resonance imaging (MRI). Am J Obstet Gynecol. 2011;204:S14. https://doi.org/10.1016/j.ajog.2010.10.033. 19. Beloosesky R, Maravi N, Weiner Z, et al. Maternal lipopolysaccharide-induced inflammation during pregnancy programs impaired offspring innate immune responses. Am J Obstet Gynecol. 2010;203:185.e1–e4. https://doi.org/10.1016/j.ajog.2010.04.033. 20. Beloosesky R, Weiner Z, Ginsberg Y, Ross MG. Maternal N-acetyl-cysteine (NAC) protects the rat fetal brain from inflammatory cytokine responses to lipopolysaccharide (LPS). J Matern Neonatal Med. 2012;25:1324–8. https://doi.org/10.3109/14767058.2011.632793. 21. Beloosesky R, Weiner Z, Khativ N, et al. Prophylactic maternal n-acetylcysteine before lipopolysaccharide suppresses fetal inflammatory cytokine responses. Am J Obstet Gynecol. 2009;200:665.e1–e5. https://doi.org/10.1016/j.ajog.2009.01.032. 22. Ginsberg Y, Lotan P, Khatib N, et al. Maternal lipopolysaccharide alters the newborn oxidative stress and C-reactive protein levels in response to an inflammatory stress. J Dev Orig Health Dis. 2012;3:358–63. https://doi.org/10.1017/S204017441200027X. 23. Lamhot VB, Khatib N, Ginsberg Y, et al. Magnesium sulfate prevents maternal inflammation-induced impairment of learning ability and memory in rat offspring. Am J Obstet Gynecol. 2015;213:851.e1–8. https://doi.org/10.1016/j.ajog.2015.07.042. 27. Burd I, Bentz AI, Chai J, et al. Inflammation-induced preterm birth alters neuronal morphology in the mouse fetal brain. J Neurosci Res. 2010;88:1872–81. https://doi.org/10.1002/jnr.22368. 31. Elovitz MA, Brown AG, Breen K, Anton L, Maubert M. Intrauterine inflammation, insufficient to induce parturition, still evokes fetal and neonatal brain injury. Int J Dev Neurosci. 2011;29:663–71. https://doi.org/10.1016/j.ijdevneu.2011.02.011. 33. Meyer U, Feldon J, Schedlowski M, Yee BK. Immunological stress at the maternal–foetal interface: a link between neurodevelopment and adult psychopathology. Brain Behav Immun. 2006;20:378–88. https://doi.org/10.1016/j.bbi.2005.11.003. 37. Huleihel M, Golan H, Hallak M. Intrauterine infection/inflammation during pregnancy and offspring brain damages: possible mechanisms involved. Reprod Biol Endocrinol. 2004;2:17. https://doi.org/10.1186/1477-7827-2-17. 39. Jurewicz A, Matysiak M, Tybor K, Kilianek L, Raine CS, Selmaj K. Tumour necrosis factor-induced death of adult human oligodendrocytes is mediated by apoptosis inducing factor. Brain. 2005;128:2675–88. https://doi.org/10.1093/brain/awh627. 40. Pang Y, Cai Z, Rhodes PG. Effect of tumor necrosis factor-alpha on developing optic nerve oligodendrocytes in culture. J Neurosci Res. 2005;80:226–34. https://doi.org/10.1002/jnr.20450. 41. Inder TE, Wells SJ, Mogridge NB, Spencer C, Volpe JJ. Defining the nature of the cerebral abnormalities in the premature infant: a qualitative magnetic resonance imaging study. J Pediatr. 2003;143:171–9. https://doi.org/10.1067/S0022-3476(03)00357-3. 43. Gayle DA, Beloosesky R, Desai M, Amidi F, Nuñez SE, Ross MG. Maternal LPS induces cytokines in the amniotic fluid and corticotropin releasing hormone in the fetal rat brain. Am J Physiol Regul Integr Comp Physiol. 2004;286:R1024–9. https://doi.org/10.1152/ajpregu.00664.2003. 44. Ejima K, Koji T, Tsuruta D, Nanri H, Kashimura M, Ikeda M. Induction of apoptosis in placentas of pregnant mice exposed to lipopolysaccharides: possible involvement of Fas/Fas ligand system. Biol Reprod. 2000;62:178–85. https://doi.org/10.1095/biolreprod62.1.178. 47. Gao F, Ding B, Zhou L, Gao X, Guo H, Xu H. Magnesium sulfate provides neuroprotection in lipopolysaccharide-activated primary microglia by inhibiting NF-κB pathway. J Surg Res. 2013;184:944–50. https://doi.org/10.1016/j.jss.2013.03.034. 48. Crowther CA, Hiller JE, Doyle LW, Haslam RR. Australasian Collaborative Trial of Magnesium Sulphate (ACTOMg SO4) Collaborative Group. Effect of magnesium sulfate given for neuroprotection before preterm birth: a randomized controlled trial. JAMA. 2003;290:2669–76. https://doi.org/10.1001/jama.290.20.2669. 49. Blackwell SC, Hallak M, Hassan SS, Berry SM, Russell E, Sorokin Y. The effects of intrapartum magnesium sulfate therapy on fetal serum interleukin-1beta, interleukin-6, and tumor necrosis factor-alpha at delivery: a randomized, placebo-controlled trial. Am J Obstet Gynecol. 2001;184:1320–3. https://doi.org/10.1067/mob.2001.115745. 50. Rouse DJ, Hirtz DG, Thom E, et al. A randomized, controlled trial of magnesium sulfate for the prevention of cerebral palsy. N Engl J Med. 2008;359:895–905. https://doi.org/10.1056/NEJMoa0801187. 53. Yoon BH, Romero R, Kim CJ, Jun JK, Gomez R, Choi J-H, Syu HC. Amniotic fluid interleukin-6: A sensitive test for antenatal diagnosis of acute inflammatory lesions of preterm placenta and prediction of perinatal morbidity. Am J Obstet Gynecol. 1995;172:960–70. https://doi.org/10.1016/0002-9378(95)90028-4. 54. Wang X, Svedin P, Nie C, et al. N-acetylcysteine reduces lipopolysaccharide-sensitized hypoxic-ischemic brain injury. Ann Neurol. 2007;61:263–71. https://doi.org/10.1002/ana.21066. |