| |
|
RMMJ
Rambam Maimonides Medical Journal
Rambam Health Care Campus 2020 April; 11(2): e0015.
ISSN: 2076-9172 Published online 2020 April 29. doi: 10.5041/RMMJ.10401 Special Issue on Cardiology in the 21st Century Honoring the Contributions of Professor Rafael Beyar with Guest Editor Lior Gepstein, M.D., Ph.D.
Clinical-based Cell Therapies for Heart Disease—Current and Future State Darren Turner, B.A.,1 Angela C. Rieger, M.S., M.D.,1 Wayne Balkan, Ph.D.,1,2 and Joshua M. Hare, M.D.1,2* 1Interdisciplinary Stem Cell Institute, University of Miami Miller School of Medicine, Miami, Florida, USA 2Department of Medicine, University of Miami Miller School of Medicine, Miami, Florida, USA
|
Patients have an ongoing unmet need for effective therapies that reverse the cellular and functional damage associated with heart damage and disease. The discovery that ~1%–2% of adult cardiomyocytes turn over per year provided the impetus for treatments that stimulate endogenous repair mechanisms that augment this rate. Preclinical and clinical studies provide evidence that cell-based therapy meets these therapeutic criteria. Recent and ongoing studies are focused on determining which cell type(s) works best for specific patient population(s) and the mechanism(s) by which these cells promote repair. Here we review clinical and preclinical stem cell studies and anticipate future directions of regenerative medicine for heart disease.
Keywords: Cell therapy, ischemic cardiomyopathy, non-ischemic cardiomyopathy, stem cells |
The leading cause of death by non-communicable disease in the world is cardiovascular disease (CVD), and the American Heart Association estimates that over half of all Americans above the age of 40 suffer from CVD, much of it hypertension-related.1 While the number of Americans dying from CVD was decreasing, that trend began reversing in ~2012.1 Current treatments for CVD focus primarily on slowing disease progression or ameliorating pre-existing myocardial damage; however, the field lacks interventions that fundamentally reverse the progressive nature of CVD. Many patients with end-stage heart damage will require a heart transplant,1 yet there is a profound shortage of donors,2 illustrating the tremendous need for alternative/novel therapies.
One such approach is stem cell or cell-based therapy, a relatively new frontier in biomedical research that has sparked much debate and controversy in cardiovascular medicine.3,4 The heart was once thought to be incapable of regeneration, but the current consensus is that ~1%–2% of cardiomyocytes turn over each year with a rate that decreases with age. Cardiac remodeling has been characterized by a persistent inflammatory reaction after acute stress and during chronic pathologies,5 increased oxidative stress,5 myocyte apoptosis,6 imbalanced oxygen consumption, energy metabolism and extracellular matrix formation contributing to scar formation,7 endothelial dysfunction,8 and decreased capillary density and neovascularization.9 Stem cells and other cell-based therapies hold promise to counteract these effects and promote cardiac repair. Stem cells, strictly defined, possess the properties of both self-renewal and differentiation, whereas other cell-based approaches act through the transmission of factors that stimulate endogenous regenerative pathways. Current data support the idea that both approaches improve cardiac structure and function, and this implication of cardiac repair has spurred much excitement in the field.10 A current great debate is whether engraftment and differentiation of exogenously administered pluripotent stem cells is a requirement for a therapeutic response, with some investigators arguing that it is an essential requirement for a therapeutic response.11,12 This controversy is intensified by the observation that pluripotent stem cell therapies produce ventricular arrhythmias in preclinical, large-animal models,11,13,14 delaying clinical testing and the ability to compare these approaches with non-pluripotent cell-based therapies, which enjoy a substantial safety profile.
Clinical trials have assessed the safety and feasibility of cell-based therapy, largely testing culture-expanded cells from bone marrow, adipose tissue, or the heart itself. While initial studies demonstrated positive results, some trials have produced little or no functional improvements in cardiac performance. A majority of studies have focused on surrogate primary end points, such as changes in left ventricular ejection fraction (LVEF) and cardiac volumes, but in some studies only small improvements (5% on average) were seen, which has dampened enthusiasm toward the field.15–17 However, substantial efforts continue toward improving cell-based approaches for cardiac repair. Here, we will review clinical trials of cell-based therapy for heart disease and speculate on potential future directions of regenerative cardiovascular medicine. |
As mentioned above, a debate currently exists as to whether cell engraftment and differentiation is a requirement for a therapeutic response. Existing data suggest that functional and clinical responses can result from cell therapy using cell types that lack significant myocyte differentiation capacity.18–20 However, there is little evidence that stem cells engraft into the target tissue in the long term, suggesting a primarily paracrine mechanism of action.21,22 Indeed, secretions of exosomes, growth factors, cytokines, and metalloproteinases are mechanisms that contribute to the regenerative capacity of cells.21–23 Stem cells also interact with host cells via heterocellular coupling, wherein the cells communicate directly through gap junctions and tunneling nanotubes to transfer small molecules and mitochondria, respectively.21,24 However, a lack of a complete understanding of the mechanisms involved should not preclude clinical studies for evaluating efficacy.
Initial or first-generation stem cells were/are derived from adult tissues, such as those isolated by bone marrow aspiration, and comprise either mixtures of different progenitor cell types, such as unfractionated bone marrow-derived mononuclear cells (BMMNCs), a heterogeneous population of stem cells, or more pure stem cell populations, many of which were isolated from BMMNCs (Figure 1). This latter group of cells includes hematopoietic stem cells (HSCs), mesenchymal stem cells (MSCs), endothelial progenitor cells (EPCs), and others.26 Mesenchymal stem cells, initially isolated from bone marrow, have been isolated from multiple tissues including adipose tissue, dental pulp, placenta, umbilical cord blood, and Wharton’s jelly.27,28 Mesenchymal stem cells exhibit properties important for a reparative cell, including immunomodulation29,30 and anti-fibrotic,31 proangiogenic, and anti-oxidative effects, all of which provide support for their being ideal candidates for treatment of cardiomyopathies. Furthermore, the lack of MHC class antigens confers immunoprivileged characteristics that make MSCs suitable for allogeneic therapy.18 Endothelial progenitor cells are primarily bone marrow-derived circulating progenitor cells characterized by the surface markers CD34 and CD133.26 Endothelial progenitor cell characteristics associated with their regenerative capacity include migration to injured areas to restore the endothelial niche, their proangiogenic properties, and ability to improve endothelial function.32 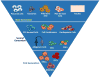 | Figure 1 Advances in Cardio-regenerative Medicine |
Second-generation cell therapies include cardiac-committed progenitor cells, that are lineage-directed by either genetic or secondary modifications to acquire a specific phenotype, or pluripotent stem cells (PSCs). Cardiac-committed progenitor cells include c-kit+ cardiac stem cells (CSCs). These clonogenic and multipotent cells can differentiate under secondary injury, contributing to organ regeneration. In vitro, CSCs display strong paracrine signaling and multilineage transdifferentiation, making them suitable for cardiac regeneration.33 Cardiosphere-derived cells (CDCs) are a heterogeneous population of cells isolated from myocardial tissue; they comprise CSCs and support cells and are capable of forming self-adherent clusters in vitro.34,35 These cells exhibit multilineage as well as clonogenic characteristics. Cardiopoietic cells are lineage-directed MSCs treated with multiple growth factors to bolster their stemness potential and differentiation.36
The PSCs (embryonic stem cells [ESCs] and induced pluripotent stem cells [iPSCs]) have the greatest multilineage capabilities37–39; however, the risk of teratoma formation requires that these cells first undergo lineage-directed differentiation prior to transplantation.40,41 Additional post-transplantation concerns include the risk of arrhythmias11,13,14 and rejection by the recipient. In preclinical studies these cells have demonstrated variable effects on the restoration of cardiac function.11,13,14
Third-generation therapy includes genetic reprogramming, exosomes, microRNA (miRNA), and the use of biomaterials to enhance the differentiation and regenerative capabilities of the cells.40 Exosomes are extracellular bilayer membrane vesicles that contain a diverse collection of proteins, lipids, and mRNAs/miRNAs and are secreted by a multitude of cell types.42 The exosomes secreted by iPSCs, ESCs, MSCs, and CDCs have different profiles,43,44 which ultimately physiologically manifest as increased self-renewal or expansion. Moreover, there is a growing body of evidence that exosome secretion is an important mode of cardiac cell communication.21,22
Route of Delivery Several factors contribute to the success of stem cell therapy. One of the most significant factors is the route of delivery 45,46; yet there is no consensus on the best route. There are four primary methods of administration that are clinically practical, and each has its own advantages and disadvantages ( Figure 2). For instance, although intracoronary delivery may cause poor cell retention in the heart, it carries the benefit of minimal inflammation. 47 Transendocardial stem cell injections (TESI) are a minimally invasive technique where stem cells are injected directly into the myocardium through the endocardium. This procedure carries a small risk of perforation and arrhythmias; however, the retention of the cells is higher compared to other methods and in certain pathologies has shown greater effectiveness. 45 Intravenous delivery of stem cells is the least invasive route and takes advantage of physiological attraction signals which induce cellular homing to the site of injury. 48 With intravenous administration, there are concerns of poor implantation and retention. Unfortunately, very few studies have directly compared the therapeutic difference between routes of delivery. 49 A meta-analysis of preclinical studies in models of acute myocardial infarction (AMI) by Kanelidis et al. 45 concluded that TESI was associated with improved efficacy over intracoronary delivery. Additional preclinical and clinical studies are needed to establish an optimal route of delivery, and the most efficacious route may be cell-type dependent. 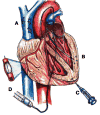 | Figure 2 Various Approaches for Stem Cell Delivery to the Heart |
Acute Myocardial Infarction Bone-marrow derived mononuclear stem cells (BMMNCs) were some of the earliest cell types used in regenerative medicine to treat AMI. 50,51 These cells are typically harvested from bone marrow and contain a heterogeneous group of cells including HSCs, MSCs, and EPCs. 52 Because these cells can be obtained from bone marrow aspiration and do not require extensive expansion, BMMNCs are ideal for use in the setting of AMI and have been assessed in over 100 studies. 53 Clinical trials involving BMMNCs were first conducted in the setting of AMI.
One of the first clinical trials testing therapeutic efficacy of BMMNCs was TOPCARE-AMI (Transplantation of Progenitor Cells and Regeneration Enhancement in Acute Myocardial Infarction).54 This study evaluated BMMNCs delivered an average of 5 days after an AMI. The trial reported a significantly increased LVEF and reduced scar size. Two years later, the BOOST (Bone Marrow Transfer to Enhance ST-Elevation Infarct Regeneration)55 trial showed similar promising results. It should be noted that the TOPCARE-AMI trial did not include a control group and the BOOST trial was open-label, since the control patients did not receive additional procedures, only standard care, compared to cell-treated patients. Another trial, LEUVEN-AMI, also reported improved LVEF after BMMNC infusion therapy.56 The REPAIR-AMI (The Reinfusion of Enriched Progenitor Cells and Infarct Remodeling in Acute Myocardial Infarction)57 trial is the largest phase III, double-blinded, placebo-controlled clinical trial to date and was conducted to assess the efficacy of BMMNCs. In this study, patients in the cell-treated arm had a significantly improved LVEF (5.5% in BMMNC group) compared to placebo (3.0%). After 1 year, death, myocardial infarction (MI), and the need for revascularization were lower in the BMMNC group.
Although the initial studies were exciting, many subsequent studies demonstrated, at best, inconclusive results. The ASTAMI (the Autologous Stem-Cell Transplantation in Acute Myocardial Infarction)58 trial, undertaken in 2006, demonstrated that after a 6-month follow-up, patients receiving cell treatment 6 days post-AMI showed no significant difference in LVEF or scar size compared to patients administered placebo. The multicenter, double-blinded, placebo-controlled TIME (Use of Adult Autologous Stem Cells in Treating People Who Have Had a Heart Attack)59 and LateTIME (Use of Adult Autologous Stem Cells in Treating People 2 to 3 Weeks After Having a Heart Attack)60 trials found no improvements in LVEF, left ventricular volumes, or wall motion as measured by cardiac magnetic resonance imaging between BMMNC and placebo groups. The SWISS AMI (Swiss Multicenter Intracoronary Stem Cells Study in Acute Myocardial Infarction)61 trial was a multicentered, open-labeled clinical trial that treated patients with BMMNCs either 5–7 days or 3–4 weeks after AMI. Neither group improved left ventricular function or scar size at 12 months; however, there was a high drop-out rate in this study. Finally, the repeat BOOST-2 trial62 was unable to replicate the results of the original study.
Preclinical data showed that a subpopulation of BMMNCs that were CD34+ could be a more suitable cell for AMI because of their angiogenic capacity.63 As such, the PreSERVE-AMI (NBS10 Versus Placebo Post ST Segment Elevation Myocardial Infarction)64 trial, the largest trial of stem cells for AMI in the United States, was conducted. This trial failed to show improvement in LVEF or resting myocardial perfusion; however, tertiary analyses demonstrated a significant association between change in LVEF and cell dose after adjusting for total ischemic time.64
The growing evidence of MSCs playing a key role in cardiac repair encouraged researchers to investigate their therapeutic efficacy in clinical trials.65 The effects of MSCs are the result of the secretion of cytokines, trophic factors, and matrix metalloproteinases which modulate the extracellular matrix and reduce infarct size and fibrosis.31 Compared to BMMNCs, human (h) MSCs are more efficacious in the setting of AMI. Hare et al.65 reported that precultured allogeneic hMSCs administered intravenously are safe, reduced episodes of ventricular tachycardia, and improved LVEF (6.7% greater than baseline). Another trial, WJ-MSC-AMI (Intracoronary Human Wharton’s Jelly-derived Mesenchymal Stem Cells Transfer in Patients with Acute Myocardial Infarction),66 demonstrated that MSCs derived from human umbilical cords increased LVEF (7.8%± 0.9% versus 2.8%±1.2%) and decreased end systolic volume (ESV) and end diastolic volume (EDV). There is also an ongoing trial, AMICI (Safety Study of Allogeneic Mesenchymal Precursor Cell Infusion in Myocardial Infarction), which is a phase II trial examining intracoronary delivery of mesenchymal precursor cells (NCT01781390).
Allogeneic CSCs have also been tested for therapeutic efficacy in the phase I/II, randomized, double-blind, placebo-controlled CAREMI trial (Cardiac Stem Cells in Patients with Acute Myocardial Infarction).67 Cardiac stem cells did not significantly improve scar size, left ventricular volumes, LVEF, or regional wall motion after 1 year of follow-up. |
CHRONIC ISCHEMIC CARDIOMYOPATHY
Despite advances in interventional care for AMI, patients frequently go on to develop chronic ischemic cardiomyopathy (ICM). The growing evidence of efficacy of stem cell treatment in AMI inspired researchers to begin experiments and clinical trials investigating stem cell therapy in chronic ICM. A major paradigm of treatment for ICM is the attenuation of left ventricular enlargement. Compared to mainstream treatment, regenerative medicine seeks to restore normal function, potentially being curative rather than palliative. As ICM is a chronic condition, researchers can utilize and cultivate a variety of cell types in an effort to maximize therapeutic effects.
There are significantly fewer studies carried out with BMMNCs in the setting of ICM. The first study exploring the effects of BMMNCs on patients with ICM was carried out by Perin et al.68 In this prospective, non-randomized, open-labeled study, BMMNCs were delivered via TESI. An evaluation performed 4 months later concluded that LVEF significantly increased from a baseline of 20% to 29%, which was accompanied by a reduction in ESV in treated patients. The TOPCARE-CHD (Transplantation of Progenitor Cells and Regeneration Enhancement in Chronic Postinfarction Heart Failure)69 trial showed small but similarly significant increases in LVEF with BMMNC treatment that correlated with reductions in N-terminal pro-brain natriuretic peptide (NT-proBNP). However, cell treatment did not reduce scar size compared to placebo. Subsequent studies were not able to replicate the positive effects on LVEF. The FOCUS-CCTRN (First Mononuclear Cells Injected in the United States conducted by the Cardiovascular Cell Therapy Research Network)70 trial, a phase II, randomized double-blind, placebo-controlled study, showed no significant difference in LVEF or infarct size in patients treated with BMMNCs.
The TAC-HFT (The Transendocardial Autologous Cells [hMSC or hBMC] in Ischemic Heart Failure Trial)71 was one of the earliest trials examining MSCs as a treatment for ICM. Importantly, it was the first trial to compare BMMNCs to MSCs head-to-head. This phase II randomized, placebo-controlled study failed to demonstrate improvements in LVEF or left ventricular volumes; however, both groups showed improvement in quality of life (QoL) as measured by the Minnesota Living with Heart Failure Questionnaire (MLHFQ) score. Additionally, the 6-minute walk distance (6MWD) improved only in the MSC group. Infarct size was reduced by 19% in the MSC group, whereas in the BMMNC and placebo groups it remained unchanged. The POSEIDON trial (Percutaneous Stem Cell Injection Delivery Effects on Neomyogenesis)72 study compared allogeneic to autologous MSCs and demonstrated that both MSC groups reduced scar size by ~33%, consistent with the TAC-HF trial. Of note, both the POSEIDON and TAC-HFT studies showed that QoL can be improved without concomitant improvements in LVEF. The MSC-HF trial73 assessed TESI of autologous MSCs and showed increased LVEF of 6.2% compared with placebo, as well as reduced left ventricular ESV. These increases were maintained at the 1-year follow-up while myocardial mass was greater than at 6 months.74 There was a correlation between cell dose and improvements.74 The randomized, double-blinded TRIDENT trial75 examined dose-dependence of allogeneic MSCs. In this study, the 100 million cell dose improved absolute LVEF by 3.6% compared to no change in the 20 million cell dose group after 12 months. Additional, larger clinical trials are needed.
A more recent approach is treatment using combinations of stem cells, which may provide greater therapeutic efficacy than a single cells type, as was observed with MSCs+CSCs in preclinical studies in porcine models of ICM.76–78 These porcine studies formed the basis of the ongoing phase II CONCERT-HF trial (Combination of Mesenchymal and C-kit+ Cardiac Stem Cells as Regenerative Therapy for Heart Failure),79 which is assessing if the combination of MSCs plus CSCs provides greater cardiac repair in humans than either cell type alone. Other clinical80 and preclinical77,81 studies have also demonstrated a positive effect of combination stem cell therapy.
Cardiospheres were first described after a population of cells isolated from subcultures of atrial or ventricular biopsy specimens were shown to be able to differentiate into cardiomyocytes, endothelial cells, and smooth muscle cells.34 Cardiospheres contain a heterogeneous mixture of cell types including cells that express endothelial (KDR [human]/flk-1 [mouse], CD31), stem cell (CD34, c-kit, Sca-1), and mesenchymal (CD105, CD90) cell surface markers.34 However, the specific cell type contributing to cardiac functioning and remodeling is unknown.82 Promising preclinical work provided the basis for a phase I, randomized trial, CADUCEUS (Cardiosphere-Derived Autologous Stem Cells to Reverse Ventricular Dysfunction),83 in patients with ICM. At 1.5–3 months after MI, 17 patients were administered intracoronary injections of autologous CDCs (98% of cells given were CD105-positive). Although scar size was reduced by 42% in the treatment arm, CDC therapy failed to increase LVEF, reduce left ventricular volumes, or improve QoL as measured by MLHFQ. The ALLSTAR (Allogeneic Heart Stem Cells to Achieve Myocardial Regeneration)84 trial using CDCs had to be suspended by the Data Safety Monitoring Board because the study failed to meet the primary end point. Given the heterogeneous nature of this cell preparation, it may be difficult to identify which cell type(s) truly contributes to any beneficial effects.
Cardiopoietic stem cells (CPSCs) are MSCs that are manipulated ex vivo to undergo cardiopoiesis in order to enhance their cardio-reparative functionality.85,86 The randomized, double-blinded, placebo-controlled CHART-1 (Congestive Heart Failure Cardiopoietic Regenerative Therapy)87 trial was conducted to ascertain the safety and therapeutic value of CPSCs in patients with ICM. The primary efficacy end point of a Finkelstein–Schoenfeld hierarchical composite (mortality, worsening heart failure, MLHFQ, 6MWD, LVEF, ESV) at 39 weeks was not achieved. |
NON-ISCHEMIC DILATED CARDIOMYOPATHY
Non-ischemic dilated cardiomyopathy (NIDCM) is the leading cause of death among heart transplant recipients.88 As with ICM, BMMNCs were the first cell type to be tested in the setting of NIDCM. In the TOPCARE-DCM (Transplantation of Progenitor Cells and Recovery of Left Ventricular Function in Patients with Non-Ischemic Dilatative Cardiomyopathy)89 trial, patients showed improvements in LVEF, regional wall motion at 3 months after treatment, and decreased NT-proBNP levels at 1-year follow-up. Similarly, the ABCD (Autologous Bone Marrow Cells in Dilated Cardiomyopathy)90 trial found positive results, including QoL parameters, which conflicted with the MiHeart,91 a multicenter, randomized, double-blind clinical trial that evaluated intracoronary delivery of BMMNCs and showed no significant changes in LVEF and left ventricular volumes.
Compared to ICM, NIDCM has a more significant immunologic component.92 As such, MSC ther-apy could prove beneficial due to its immunomodulatory, reverse remodeling, and regenerative properties.93,94 The POSEIDON-DCM trial (Percutaneous Stem Cells Injection Delivery Effects on Neomyogenesis in Dilated Cardiomyopathy)25 randomly allocated 37 patients with idiopathic NIDCM to receive TESI of allogeneic or autologous MSCs. Functional parameters and LVEF increased significantly only in the allogeneic group (Figure 3). Of note, LVEF increases were not accompanied by reductions in left ventricular volumes, suggesting that reverse remodeling is not the primary means by which cardiac function is improved. Incidence of major adverse cardiac events and hospitalization rate was also significantly lower in the allogeneic group.25 Moreover, treatment with allogenic MSCs significantly increased QoL and functional capacity. Both treatment arms noted significantly decreased systemic tumor necrosis factor (TNF)-α levels. The POSEIDON-DCM trial also demonstrated that patients lacking a pathologic genetic variant responded better to cell therapy (Figure 4).96 However, this study lacked a control group, and this approach should be further investigated in a larger study. A study by Vertelov et al. observed that ischemia-tolerant MSCs, i.e. hMSCs cultured under hypoxic conditions, are more therapeutically efficacious than hMSCs grown in normoxia.97 To ascertain this effect in vivo, Butler et al.98 conducted a pilot study in which bone marrow-derived MSCs isolated from healthy donors were grown under hypoxic conditions and subsequently administered to 22 patients. Although no improvements in left ventricle anatomy or function were noted, QoL and 6MWD scores improved significantly in the treatment arm. 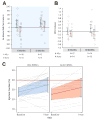 | Figure 3 Differences in the Therapeutic Efficacy of Allogeneic hMSC and Autologous hMSC |
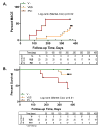 | Figure 4 Genetic Variation Affects Major Adverse Clinical Events (MACE) and Survival in Response to Delivery of MSCs |
Interestingly, the administration of CD34+ cells demonstrated consistent improvements in LVEF,99 6MWD, brain natriuretic peptide (BNP) levels, as well as survival at 1 and 5 years post-treatment.99 The comparison between intra-coronary and TESI delivery demonstrated that TESI produced higher engraftment and therapeutic efficacy.49 Importantly, a subset of patients with NIDCM and diabetes mellitus did not respond similarly to the non-diabetic population, which had an improvement in LVEF.100 These studies demonstrate that specific subpopulations of patients respond to a greater or lesser extent to the same therapy, illustrating the importance of adequately assessing the profile of patients, the cell(s) to be delivered, and the route of delivery. |
OTHER POTENTIAL SOURCES OF CELLS FOR CARDIAC REPAIR
Pluripotent Stem Cells While many stem cells have been tested for their cardio-reparative capacity, ESCs and iPSCs have yet to be thoroughly assessed in clinical trials. As mentioned above, these cells have the greatest multilineage capability, but also some of the highest potential risks. 37–39,101
Embryonic Stem Cells Embryonic stem cells are immortal, pluripotent cells derived from the inner cell mass of the pre-implantation embryo, that are propagated ex vivo. 102,103 However, the risk of teratoma formation requires that these cells first undergo lineage-directed differentiation prior to transplantation. 40,41 Additional concerns after transplantation are the risk of arrhythmias and rejection by the recipient.
Two preclinical studies involved the intramyocardial administration of human ESC-derived cardiomyocytes (hESC-CMs) into non-human primates following ischemia/reperfusion injury.11,13 Pigtail macaques were kept immunosuppressed and administered 1×109 hESC-CMs 2 weeks post-MI11 or 750×108 hESC-CMs 4 weeks post-MI.13 The hearts exhibited islands of engrafted hESC-CMs, but there was no reduction of infarct size, and non-lethal ventricular arrhythmias were seen in all animals.11,13 A similar study was conducted using 1×109 hESC-CMs in a porcine model of MI. Similar to the macaque studies, the immunosuppressed pigs had islands of engrafted hESC-CMs but no cardiac functional or structural improvements (Figure 5).14 These results suggest that further preclinical studies are needed to optimize the therapeutic effects of hESC-CMs. However, Menasche et al. demonstrated that hESC-derived cardiac progenitor cells embedded into a fibrin scaffold are safe in a patient with severe heart failure.41 Cell therapy did not cause complications, and after 3 months the patient showed improvements in cardiac function. Importantly, the European clinical study, ESCORT, in which hESC-derived cardiac progenitors were transplanted within a fibrin patch into heart failure patients (n=6), showed safety and efficacy.41 One patient died early post-operation from treatment-unrelated comorbidities. The other 5 patients showed no evidence of significant adverse effects (SAEs), and they improved symptomatically with an improved wall motion of the cell-treated segments. 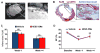 | Figure 5 In a Porcine Model of MI, hESC-CMs Demonstrated Engraftment but no Functional or Structural Improvements |
Induced Pluripotent Stem Cells Due to the ethical concerns of harvesting ESCs, scientists have sought alternative methods to isolate multipotent stem cells. Takahashi and Yamanaka developed a novel protocol to generate pluripotency from murine somatic cell by integrating a variety of transcription factors into the cell’s genome via retroviral transduction. 104 This technique was then applied to human somatic cells. 105 Subsequent studies have demonstrated that these iPSCs have the capacity to differentiate into all three germ layers in addition to somatic cells, including cardiomyocytes and other cardiovascular cells. 106,107 Furthermore, these cells could also aid in repair of heart valves and vessels. 108 A major concern when using these pluripotent cells, as with ESCs, is tumorigenesis. However, this risk can be mitigated by isolating cells or cell lines that have undergone at least some differentiation. 109 An initial clinical trial to evaluate safety and efficacy of a patch with 100 million reprogrammed iPSC cardiomyocytes was approved in Japan. Three patients with ICM were treated initially; a further 7–10 patients will be recruited and followed up over the period of 1 year. 110 The Treating Heart Failure With hPSC-CMs (HEAL-CHF) Trial (NCT03763136) is an open-label study recruiting 5 patients to receive epicardial injection of allogeneic PSC-CMs. There are as yet no reports from either of these two studies. Continuing studies will have to investigate methods to maintain stable cell lines as well as address scalability for clinical grade production. 111 Table 1 compares the efficacy of different cell types for increasing LVEF, and reducing EDV and scar size in clinical trials to date.
Placental Stem Cells The placenta is a novel source of potentially cardio-regenerative cells. Perinatal tissue is a rich source of a variety of stem cells that can be isolated from the amnion, chorion, umbilical cord (e.g. Wharton’s jelly) and the placental cotyledons from the fetal side and the decidua from the maternal side. Many of these cells display MSC-like characteristics, such as adherence to plastic and immunomodulation. Furthermore, in vitro, they inhibit cardiomyocyte apoptosis and are pro-angiogenic (reviewed by Bollini et al. 117).
Cells isolated from the murine near-term placenta and expressing the Caudal-type homeobox-2 (Cdx2) were recently reported to form beating cardiomyocytes and vascular lineages ex vivo. Furthermore, these Cdx2+ cells homed to the injured heart and promoted cardiac repair when injected intravenously (1×106 cells) post-MI in a mouse model. Three months post-injection, the cells were found integrated within the myocardium, primarily in the border zone, where they exhibited a cardiomyocyte morphology. Cell-treated hearts exhibited improved LVEF and stroke volume and reduced adverse remodeling compared to placebo-injected mice.118
Tissue-specific MSCs Most studies have assessed the therapeutic effects of bone marrow- and adipose tissue-derived MSCs. These cells can be isolated and expanded in large quantities while retaining their immunomodulatory characteristics, 119 but the properties of these MSCs are influenced by their tissue of origin. For example, bone marrow-derived MSCs are highly proangiogenic 120 and may be more immunosuppressive than adipose-derived MSCs. 121,122 Mesenchymal stem cells have also been isolated from other tissues, including umbilical cord (Wharton’s jelly), amniotic fluid, peripheral blood, and the heart. Again, the tissue of origin appears to provide MSCs with characteristic properties 122–126 and secretomes, 127 and for therapeutic use it may be important to determine which MSC source is best for a specific patient. |
Some studies suggest that exosomes have an (almost) equivalent therapeutic efficacy as intact cells.128–130 Other data also demonstrate that the therapeutic effect of cell therapy may not correlate with engraftment,131 supporting a paracrine mechanism. The discovery of these paracrine mechanisms of repair not only significantly challenges the notion of engraftment-dependent healing, but also opens another avenue of therapy delivery.42,132 Engineered exosomes with an ischemic myocardium-targeting peptide can enhance myocardial viability and reduce infarct size after MI in mouse models.43,133–135 Cell-free suspensions containing important reparative exosomes could be used instead of intact cells, avoiding some of the inherent issues associated with cells, such as ex vivo expansion, tumor formation, and immune rejection. Studies directly comparing the different approaches will provide guidance toward the most therapeutic approach. |
PATCHES/BIOMATERIALS: BIOENGINEERING IN STEM CELL THERAPY
Transplantation of viable cells into the harsh environment of necrotic myocardium remains a significant therapeutic challenge resulting in very poor cell retention.136,137 To combat this problem, tissue engineering approaches have designed biomaterials as cell retention mediums. These injectable biomaterials must perform many (often contradictory) functions. They must be biodegradable, biocompatible, provide mechanical support, be of appropriate dimension, allow for precise placement,138 improve cell survival, and promote tissue regeneration.139,140 These polymers can either be synthetic or naturally derived, each having their own advantages and disadvantages. Some polymers can even be specifically tailored to optimize cardiac repair,141 and 3D-printing has increased the available types of biomaterials, improving cell integration and vascularization.142 Preclinical studies have demonstrated improved cell viability and cardiac repair when used with human pluripotent stem cells and MSCs.141,143,144 While significant progress has been made, improving polymer compatibility and mechanical properties must occur before clinical studies can begin. |
Stem cell and cell-based therapy is still relatively new, and studies need to define the cell type/cell product, the frequency and route of stem cell injection, and the patient population most likely to respond. Recent preclinical studies show that the administration of a large number of exosomes often produces similar cardiac repair as cell injection,145,146 prompting the view that the cells are not needed. However, this equivalency is often dependent on the route of exosome administration and has only been demonstrated in the short term, while stem cell therapy has demonstrated long-term effects, despite poor stem cell retention and survival. Studies comparing the long-term effects of cells versus exosomes (or combination of the two) still need to be performed.
Other approaches toward optimizing stem cell therapy include assessing the effects of multiple rounds of injections. Tokita et al. demonstrated that three rounds of cardiac progenitor cell injections provided greater cardiac repair than a single injection of 3-fold more cells in a mouse model of ischemia.147 As mentioned above, the administration of a combination of stem cells is therapeutically synergistic, providing greater benefits than the individual cells in swine models of ICM,74–76 and this approach forms the basis of the CONCERT-HF clinical trial.77
Additionally, the choice of patient is important. While the CHART-1 study did not meet its primary end point, a subpopulation of patients responded well.36,85 Precision medicine approaches may also influence a patient’s responsiveness to stem cell therapy. As mentioned above, Rieger et al. recently showed that, in the POSEIDON-DCM trial, patients who did not have a specific genetic variant responded better to treatment.96 Furthermore, sex,148 age,149 and serum concentration of a variety of factors150 may play significant roles in a patient’s response and need to be taken into account when designing clinical trials (Figure 6). 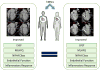 | Figure 6 Effectiveness of MSCs Is Independent of Sex of the Patient |
Clinical trials will also need to be nimble and develop better ways to assess efficacy and increase study power by incorporating ongoing results as well as new information that becomes available after the trial commences. The DREAM-HF (Double-Blind Randomized Assessment of Clinical Events With Allogeneic Mesenchymal Precursor Cells in Advanced Heart Failure; NCT02032004) is such a trial. It is a phase III, randomized, placebo-controlled study assessing the safety and efficacy of mesenchymal precursor cells (MPCs) as immunotherapy in patients with advanced, chronic heart failure with reduced ejection fraction. The DREAM-HF trial uses patient enrichment strategies to establish a patient population with reduced heterogeneity (baseline disease enrichment), high targeted outcome event rate (prognostic enrichment) and higher likelihood to respond (predictive enrichment). Adaptive statistical models are also needed. The DREAM-HF trial uses a joint frailty model, which treats terminal and recurrent heart failure events differently and models correlations between recurrent and terminal events, which takes into account random, between-patient differences. Such innovative approaches will allow for smaller, yet more definitive trial designs.151 Along with CONCERT-HF, DREAM-HF is likely to report results in 2020, and together these trials will add substantially to the clinical and mechanistic data base of the potential of cell-based therapy for chronic heart failure. |
The past two decades have witnessed substantial translational efforts to develop cell-based therapies for heart disease. While many clinical trials have been conducted, testing several strategies, the field has yet to yield a clear understanding of the clinical application in this important area. Nonetheless, the studies conducted to date have laid a robust groundwork for ongoing new efforts—including phase III and larger-powered phase II studies, as well as major progress at the bench and in preclinical models in the application of pluripotent stem cells. With these ongoing avenues of research, the field is moving closer to yielding a successful strategy for addressing one of the largest unmet needs in modern medicine, that of chronic heart disease. |
This work was funded by grants, R01 HL107110, R01 HL134558, 5UM 1HL113460, and R01 HL137355 from the National Institutes of Health (NIH), W81XWH-19-PRMRP-CTA from the Department of Defense (DOD) and by the Marcus Foundation, the Soffer Family and the Starr Foundation. |
AMI |
acute myocardial infarction |
BMMNCs |
bone marrow-derived mononuclear stem cells |
BNP |
brain natriuretic peptide |
CDCs |
cardiosphere-derived cells |
CPSCs |
cardiopoietic stem cells |
CSCs |
cardiac stem cells |
CVD |
cardiovascular disease |
EDV |
end diastolic volume |
EPCs |
endothelial progenitor cells |
ESCs |
embryonic stem cells |
ESV |
end systolic volume |
HSCs |
hematopoietic stem cells |
iPSCs |
induced pluripotent stem cells |
ICM |
ischemic cardiomyopathy |
LVEF |
left ventricular ejection fraction |
MI |
myocardial infarction |
MSCs |
mesenchymal stem cells |
NIDCM |
non-ischemic dilated cardiomyopathy |
QoL |
quality of life |
6MWD |
6-minute walk distance |
TESI |
transendocardial stem cell injection |
|
|
2. Sorabella RA, Guglielmetti L, Kantor A, et al. Cardiac donor risk factors predictive of short-term heart transplant recipient mortality: an analysis of the United Network for Organ Sharing database. Transplant Proc. 2015;47:2944–51. https://doi.org/10.1016/j.transproceed.2015.10.021. 5. Kalra DK, Zhu X, Ramchandani MK, et al. Increased myocardial gene expression of tumor necrosis factor-alpha and nitric oxide synthase-2: a potential mechanism for depressed myocardial function in hibernating myocardium in humans. Circulation. 2002;105:1537–40. https://doi.org/10.1161/01.cir.0000013846.72805.7e. 6. Asahara T, Masuda H, Takahashi T, et al. Bone marrow origin of endothelial progenitor cells responsible for postnatal vasculogenesis in physiological and pathological neovascularization. Cir Res. 1999;85:221–8. https://doi.org/10.1161/01.res.85.3.221. 7. Kandalam V, Basu R, Moore L, et al. Lack of tissue inhibitor of metalloproteinases 2 leads to exacerbated left ventricular dysfunction and adverse extracellular matrix remodeling in response to biomechanical stress. Circulation. 2011;124:2094–105. https://doi.org/10.1161/CIRCULATIONAHA.111.030338. 8. Kubo SH, Rector TS, Bank AJ, Williams RE, Heifetz SM. Endothelium-dependent vasodilation is attenuated in patients with heart failure. Circulation. 1991;84:1589–96. https://doi.org/10.1161/01.cir.84.4.1589. 10. Madonna R, Van Laake LW, Davidson SM, et al. Position paper of the European Society of Cardiology Working Group Cellular Biology of the Heart: cell-based therapies for myocardial repair and regeneration in ischemic heart disease and heart failure. Eur Heart J. 2016;37:1789–98. https://doi.org/10.1093/eurheartj/ehw113. 11. Chong JJ, Yang X, Don CW, et al. Human embryonic-stem-cell-derived cardiomyocytes regenerate non-human primate hearts. Nature. 2014;510:273–7. https://doi.org/10.1038/nature13233. 13. Liu YW, Chen B, Yang X, et al. Human embryonic stem cell–derived cardiomyocytes restore function in infarcted hearts of non-human primates. Nat Biotechnol. 2018;36:597–605. https://doi.org/10.1038/nbt.4162. 14. Romagnuolo R, Masoudpour H, Porta-Sanchez A, et al. Human embryonic stem cell-derived cardiomyocytes regenerate the infarcted pig heart but induce ventricular tachyarrhythmias. Stem Cell Reports. 2019;12:967–81. https://doi.org/10.1016/j.stemcr.2019.04.005. 15. Fisher SA, Doree C, Mathur A, Taggart DP, Martin-Rendon E. Stem cell therapy for chronic ischaemic heart disease and congestive heart failure. Cochrane Database Syst Rev. 2016;12:CD007888. https://doi.org/10.1002/14651858.CD007888.pub3. 17. Afzal MR, Samanta A, Shah ZI, et al. Adult bone marrow cell therapy for ischemic heart disease: evidence and insights from randomized controlled trials. Circ Res. 2015;117:558–75. https://doi.org/10.1161/CIRCRESAHA.114.304792. 18. Golpanian S, Wolf A, Hatzistergos KE, Hare JM. Rebuilding the damaged heart: mesenchymal stem cells, cell-based therapy, and engineered heart tissue. Physiol Rev. 2016;96:1127–68. https://doi.org/10.1152/physrev.00019.2015. 21. Gnecchi M, He H, Liang OD, et al. Paracrine action accounts for marked protection of ischemic heart by Akt-modified mesenchymal stem cells. Nat Med. 2005;11:367–8. https://doi.org/10.1038/nm0405-367. 22. Sluijter JP, Verhage V, Deddens JC, van den Akker F, Doevendans PA. Microvesicles and exosomes for intracardiac communication. Cardiovasc Res. 2014;102:302–11. https://doi.org/10.1093/cvr/cvu022. 24. Mayourian J, Cashman TJ, Ceholski DK, et al. Experimental and computational insight into human mesenchymal stem cell paracrine signaling and heterocellular coupling effects on cardiac contractility and arrhythmogenicity. Circ Res. 2017;121:411–23. https://doi.org/10.1161/CIRCRESAHA.117.310796. 25. Hare JM, DiFede DL, Rieger AC, et al. Randomized comparison of allogeneic versus autologous mesenchymal stem cells for nonischemic dilated cardiomyopathy: POSEIDON-DCM Trial. J Am Coll Cardiol. 2017;69:526–37. https://doi.org/10.1016/j.jacc.2016.11.009. 26. Sanganalmath SK, Bolli R. Cell therapy for heart failure: a comprehensive overview of experimental and clinical studies, current challenges, and future directions. Circ Res. 2013;113:810–34. https://doi.org/10.1161/CIRCRESAHA.113.300219. 28. Golpanian S, Schulman IH, Ebert RF, et al. Concise review: review and perspective of cell dosage and routes of administration from preclinical and clinical studies of stem cell therapy for heart disease. Stem Cells Transl Med. 2016;5:186–91. https://doi.org/10.5966/sctm.2015-0101. 31. Mirotsou M, Jayawardena TM, Schmeckpeper J, Gnecchi M, Dzau VJ. Paracrine mechanisms of stem cell reparative and regenerative actions in the heart. J Mol Cell Cardiol. 2011;50:280–9. https://doi.org/10.1016/j.yjmcc.2010.08.005. 32. Hill JM, Zalos G, Halcox JP, et al. Circulating endothelial progenitor cells, vascular function, and cardiovascular risk. N Engl J Med. 2003;348:593–600. https://doi.org/10.1056/NEJMoa022287. 35. Li TS, Cheng K, Malliaras K, et al. Direct comparison of different stem cell types and subpopulations reveals superior paracrine potency and myocardial repair efficacy with cardiosphere-derived cells. J Am Coll Cardiol. 2012;59:942–53. https://doi.org/10.1016/j.jacc.2011.11.029. 36. Bartunek J, Davison B, Sherman W, et al. Congestive Heart Failure Cardiopoietic Regenerative Therapy (CHART-1) trial design. Eur J Heart Fail. 2016;18:160–8. https://doi.org/10.1002/ejhf.434. 40. Cambria E, Pasqualini FS, Wolint P, et al. Translational cardiac stem cell therapy: advancing from first-generation to next-generation cell types. NPJ Regen Med. 2017;2:17. https://doi.org/10.1038/s41536-017-0024-1. 41. Menasche P, Vanneaux V, Hagege A, et al. Transplantation of human embryonic stem cell-derived cardiovascular progenitors for severe ischemic left ventricular dysfunction. J Am Coll Cardiol. 2018;71:429–38. https://doi.org/10.1016/j.jacc.2017.11.047. 43. Wang Y, Zhang L, Li Y, et al. Exosomes/microvesicles from induced pluripotent stem cells deliver cardioprotective miRNAs and prevent cardiomyocyte apoptosis in the ischemic myocardium. Int J Cardiol. 2015;192:61–9. https://doi.org/10.1016/j.ijcard.2015.05.020. 45. Kanelidis AJ, Premer C, Lopez J, Balkan W, Hare JM. Route of delivery modulates the efficacy of mesenchymal stem cell therapy for myocardial infarction: a meta-analysis of preclinical studies and clinical trials. Circ Res. 2017;120:1139–50. https://doi.org/10.1161/CIRCRESAHA.116.309819. 46. Kawamura M, Miyagawa S, Fukushima S, et al. Enhanced survival of transplanted human induced pluripotent stem cell-derived cardiomyocytes by the combination of cell sheets with the pedicled omental flap technique in a porcine heart. Circulation. 2013;128(11 Suppl 1):S87–94. https://doi.org/10.1161/CIRCULATIONAHA.112.000366. 47. Poynter JA, Herrmann JL, Manukyan MC, et al. Intracoronary mesenchymal stem cells promote postischemic myocardial functional recovery, decrease inflammation, and reduce apoptosis via a signal transducer and activator of transcription 3 mechanism. J Am Coll Surg. 2011;213:253–60. https://doi.org/10.1016/j.jamcollsurg.2011.04.005. 48. Rasmussen JG, Frobert O, Holst-Hansen C, et al. Comparison of human adipose-derived stem cells and bone marrow-derived stem cells in a myocardial infarction model. Cell Transplant. 2014;23:195–206. https://doi.org/10.3727/096368912X659871. 49. Vrtovec B, Poglajen G, Lezaic L, et al. Comparison of transendocardial and intracoronary CD34+ cell transplantation in patients with nonischemic dilated cardiomyopathy. Circulation. 2013;128(11 Suppl 1):S42–9. https://doi.org/10.1161/CIRCULATIONAHA.112.000230. 52. Astori G, Soncin S, Lo Cicero V, et al. Bone marrow derived stem cells in regenerative medicine as advanced therapy medicinal products. Am J Transl Res. 2010;2:285–95. 54. Schachinger V, Assmus B, Britten MB, et al. Transplantation of progenitor cells and regeneration enhancement in acute myocardial infarction: final one-year results of the TOPCARE-AMI Trial. J Am Coll Cardiol. 2004;44:1690–9. https://doi.org/10.1016/j.jacc.2004.08.014. 55. Meyer GP, Wollert KC, Lotz J, et al. Intracoronary bone marrow cell transfer after myocardial infarction: 5-year follow-up from the randomized-controlled BOOST trial. Eur Heart J. 2009;30:2978–84. https://doi.org/10.1093/eurheartj/ehp374. 56. Janssens S, Dubois C, Bogaert J, et al. Autologous bone marrow-derived stem-cell transfer in patients with ST-segment elevation myocardial infarction: double-blind, randomised controlled trial. Lancet. 2006;367:113–21. https://doi.org/10.1016/S0140-6736(05)67861-0. 57. Schachinger V, Erbs S, Elsasser A, et al. Improved clinical outcome after intracoronary administration of bone-marrow-derived progenitor cells in acute myocardial infarction: final 1-year results of the REPAIR-AMI trial. Eur Heart J. 2006;27:2775–83. https://doi.org/10.1093/eurheartj/ehl388. 58. Lunde K, Solheim S, Aakhus S, Arnesen H, Abdelnoor M, Forfang K. Autologous stem cell transplantation in acute myocardial infarction: the ASTAMI randomized controlled trial. Intracoronary transplantation of autologous mononuclear bone marrow cells, study design and safety aspects. Scand Cardiovasc J. 2005;39:150–8. https://doi.org/10.1080/14017430510009131. 59. Traverse JH, Henry TD, Pepine CJ, et al. TIME trial: effect of timing of stem cell delivery following ST-elevation myocardial infarction on the recovery of global and regional left ventricular function: final 2-year analysis. Circ Res. 2018;122:479–88. https://doi.org/10.1161/CIRCRESAHA.117.311466. 60. Traverse JH, Henry TD, Vaughan DE, et al. LateTIME: a phase-II, randomized, double-blinded, placebo-controlled, pilot trial evaluating the safety and effect of administration of bone marrow mononuclear cells 2 to 3 weeks after acute myocardial infarction. Tex Heart Inst J. 2010;37:412–20. 61. Surder D, Schwitter J, Moccetti T, et al. Cell-based therapy for myocardial repair in patients with acute myocardial infarction: rationale and study design of the SWiss multicenter Intracoronary Stem cells Study in Acute Myocardial Infarction (SWISS-AMI). Am Heart J. 2010;160:58–64. https://doi.org/10.1016/j.ahj.2010.03.039. 62. Wollert KC, Meyer GP, Muller-Ehmsen J, et al. Intracoronary autologous bone marrow cell transfer after myocardial infarction: the BOOST-2 randomised placebo-controlled clinical trial. Eur Heart J. 2017;38:2936–43. https://doi.org/10.1093/eurheartj/ehx188. 63. Chong MS, Ng WK, Chan JK. Concise review: endothelial progenitor cells in regenerative medicine: applications and challenges. Stem Cells Transl Med. 2016;5:530–8. https://doi.org/10.5966/sctm.2015-0227. 64. Quyyumi AA, Vasquez A, Kereiakes DJ, et al. PreSERVE-AMI: a randomized, double-blind, placebo-controlled clinical trial of intracoronary administration of autologous CD34+ cells in patients with left ventricular dysfunction post STEMI. Circ Res. 2017;120:324–31. https://doi.org/10.1161/CIRCRESAHA.115.308165. 65. Hare JM, Traverse JH, Henry TD, et al. A randomized, double-blind, placebo-controlled, dose-escalation study of intravenous adult human mesenchymal stem cells (prochymal) after acute myocardial infarction. J Am Coll Cardiol. 2009;54:2277–86. https://doi.org/10.1016/j.jacc.2009.06.055. 66. Gao LR, Chen Y, Zhang NK, et al. Intracoronary infusion of Wharton’s jelly-derived mesenchymal stem cells in acute myocardial infarction: double-blind, randomized controlled trial. BMC Med. 2015;13:162. https://doi.org/10.1186/s12916-015-0399-z. 67. Fernandez-Aviles F, Sanz-Ruiz R, Bogaert J, et al. Safety and efficacy of intracoronary infusion of allogeneic human cardiac stem cells in patients with ST-segment elevation myocardial infarction and left ventricular dysfunction. Circ Res. 2018;123:579–89. https://doi.org/10.1161/CIRCRESAHA.118.312823. 68. Perin EC, Dohmann HF, Borojevic R, et al. Transendocardial, autologous bone marrow cell transplantation for severe, chronic ischemic heart failure. Circulation. 2003;107:2294–302. 69. Assmus B, Fischer-Rasokat U, Honold J, et al. Transcoronary transplantation of functionally competent BMCs is associated with a decrease in natriuretic peptide serum levels and improved survival of patients with chronic postinfarction heart failure: results of the TOPCARE-CHD Registry. Circ Res. 2007;100:1234–41. https://doi.org/10.1161/01.RES.0000264508.47717.6b. 70. Perin EC, Willerson JT, Pepine CJ, et al. Effect of transendocardial delivery of autologous bone marrow mononuclear cells on functional capacity, left ventricular function, and perfusion in chronic heart failure: the FOCUS-CCTRN trial. JAMA. 2012;307:1717–26. https://doi.org/10.1001/jama.2012.418. 71. Heldman AW, DiFede DL, Fishman JE, et al. Transendocardial mesenchymal stem cells and mononuclear bone marrow cells for ischemic cardiomyopathy: the TAC-HFT randomized trial. JAMA. 2014;311:62–73. https://doi.org/10.1001/jama.2013.282909. 72. Hare JM, Fishman JE, Gerstenblith G, et al. Comparison of allogeneic vs autologous bone marrow-derived mesenchymal stem cells delivered by transendocardial injection in patients with ischemic cardiomyopathy: the POSEIDON randomized trial. JAMA. 2012;308:2369–79. https://doi.org/10.1001/jama.2012.25321. 73. Mathiasen AB, Qayyum AA, Jorgensen E, et al. Bone marrow-derived mesenchymal stromal cell treatment in patients with severe ischaemic heart failure: a randomized placebo-controlled trial (MSC-HF trial). Eur Heart J. 2015;36:1744–53. https://doi.org/10.1093/eurheartj/ehv136. 74. Mathiasen AB, Qayyum AA, Jørgensen E, et al. Bone marrow-derived mesenchymal stromal cell treatment in patients with ischaemic heart failure: final 4-year follow-up of the MSC-HF trial. Eur J Heart Fail. 2019 Dec 21; [Epub ahead of print]. https://doi.org/10.1002/ejhf.1700. 75. Florea V, Rieger AC, DiFede DL, et al. Dose comparison study of allogeneic mesenchymal stem cells in patients with ischemic cardiomyopathy (The TRIDENT Study). Circ Res. 2017;121:1279–90. https://doi.org/10.1161/CIRCRESAHA.117.311827. 76. Karantalis V, Suncion-Loescher VY, Bagno L, et al. Synergistic effects of combined cell therapy for chronic ischemic cardiomyopathy. J Am Coll Cardiol. 2015;66:1990–9. https://doi.org/10.1016/j.jacc.2015.08.879. 78. Williams AR, Hatzistergos KE, Addicott B, et al. Enhanced effect of combining human cardiac stem cells and bone marrow mesenchymal stem cells to reduce infarct size and to restore cardiac function after myocardial infarction. Circulation. 2013;127:213–23. https://doi.org/10.1161/CIRCULATIONAHA.112.131110. 79. Bolli R, Hare JM, March KL, et al. Rationale and design of the CONCERT-HF trial (combination of mesenchymal and c-kit(+) cardiac stem cells as regenerative therapy for heart failure). Circ Res. 2018;122:1703–15. https://doi.org/10.1161/CIRCRESAHA.118.312978. 81. Puymirat E, Geha R, Tomescot A, et al. Can mesenchymal stem cells induce tolerance to cotransplanted human embryonic stem cells? Mol Ther. 2009;17:176–82. https://doi.org/10.1038/mt.2008.208. 83. Makkar RR, Smith RR, Cheng K, et al. Intracoronary cardiosphere-derived cells for heart regeneration after myocardial infarction (CADUCEUS): a prospective, randomised phase 1 trial. Lancet. 2012;379:895–904. https://doi.org/10.1016/S0140-6736(12)60195-0. 84. Chakravarty T, Makkar RR, Ascheim DD, et al. ALLogeneic Heart STem Cells to Achieve Myocardial Regeneration (ALLSTAR) trial: rationale and design. Cell Transplant. 2017;26:205–14. https://doi.org/10.3727/096368916X692933. 85. Behfar A, Yamada S, Crespo-Diaz R, et al. Guided cardiopoiesis enhances therapeutic benefit of bone marrow human mesenchymal stem cells in chronic myocardial infarction. J Am Coll Cardiol. 2010;56:721–34. https://doi.org/10.1016/j.jacc.2010.03.066. 86. Bartunek J, Behfar A, Dolatabadi D, et al. Cardiopoietic stem cell therapy in heart failure: the C-CURE (Cardiopoietic stem Cell therapy in heart failURE) multicenter randomized trial with lineage-specified biologics. J Am Coll Cardiol. 2013;61:2329–38. https://doi.org/10.1016/j.jacc.2013.02.071. 87. Bartunek J, Terzic A, Davison BA, et al. Cardiopoietic cell therapy for advanced ischaemic heart failure: results at 39 weeks of the prospective, randomized, double blind, sham-controlled CHART-1 clinical trial. Eur Heart J. 2017;38:648–60. https://doi.org/10.1093/eurheartj/ehw543. 88. Lund LH, Khush KK, Cherikh WS, et al. The registry of the international society for heart and lung transplantation: thirty-fourth adult heart transplantation report-2017; focus theme: allograft ischemic time. J Heart Lung Transplant. 2017;36:1037–46. https://doi.org/10.1016/j.healun.2017.07.019. 89. Fischer-Rasokat U, Assmus B, Seeger FH, et al. A pilot trial to assess potential effects of selective intracoronary bone marrow-derived progenitor cell infusion in patients with nonischemic dilated cardiomyopathy: final 1-year results of the transplantation of progenitor cells and functional regeneration enhancement pilot trial in patients with nonischemic dilated cardiomyopathy. Circ Heart Fail. 2009;2:417–23. https://doi.org/10.1161/CIRCHEARTFAILURE.109.855023. 90. Seth S, Bhargava B, Narang R, et al. The ABCD (Autologous Bone Marrow Cells in Dilated Cardiomyopathy) trial a long-term follow-up study. J Am Coll Cardiol. 2010;55:1643–4. https://doi.org/10.1016/j.jacc.2009.11.070. 91. Martino H, Brofman P, Greco O, et al. Multicentre, randomized, double-blind trial of intracoronary autologous mononuclear bone marrow cell injection in non-ischaemic dilated cardiomyopathy (the dilated cardiomyopathy arm of the MiHeart study). Eur Heart J. 2015;36:2898–904. https://doi.org/10.1093/eurheartj/ehv477. 92. Bello D, Shah DJ, Farah GM, et al. Gadolinium cardiovascular magnetic resonance predicts reversible myocardial dysfunction and remodeling in patients with heart failure undergoing beta-blocker therapy. Circulation. 2003;108:1945–53. https://doi.org/10.1161/01.CIR.0000095029.57483.60. 94. Seropian IM, Toldo S, Van Tassell BW, Abbate A. Anti-inflammatory strategies for ventricular remodeling following ST-segment elevation acute myocardial infarction. J Am Coll Cardiol. 2014;63:1593–603. https://doi.org/10.1016/j.jacc.2014.01.014. 96. Rieger AC, Myerburg RJ, Florea V, et al. Genetic determinants of responsiveness to mesenchymal stem cell injections in non-ischemic dilated cardiomyopathy. EBioMedicine. 2019;48:377–85. https://doi.org/10.1016/j.ebiom.2019.09.043. 97. Vertelov G, Kharazi L, Muralidhar MG, Sanati G, Tankovich T, Kharazi A. High targeted migration of human mesenchymal stem cells grown in hypoxia is associated with enhanced activation of RhoA. Stem Cell Res Ther. 2013;4:5. https://doi.org/10.1186/scrt153. 98. Butler J, Epstein SE, Greene SJ, et al. Intravenous allogeneic mesenchymal stem cells for nonischemic cardiomyopathy: safety and efficacy results of a phase II-A randomized trial. Circ Res. 2017;120:332–40. https://doi.org/10.1161/CIRCRESAHA.116.309717. 99. Vrtovec B, Poglajen G, Lezaic L, et al. Effects of intracoronary CD34+ stem cell transplantation in nonischemic dilated cardiomyopathy patients: 5-year follow-up. Circ Res. 2013;112:165–73. https://doi.org/10.1161/CIRCRESAHA.112.276519. 100. Vrtovec B, Sever M, Jensterle M, et al. Efficacy of CD34+ stem cell therapy in nonischemic dilated cardiomyopathy is absent in patients with diabetes but preserved in patients with insulin resistance. Stem Cells Transl Med. 2016;5:632–8. https://doi.org/10.5966/sctm.2015-0172. 101. Kehat I, Kenyagin-Karsenti D, Snir M, et al. Human embryonic stem cells can differentiate into myocytes with structural and functional properties of cardiomyocytes. J Clin Invest. 2001;108:407–14. https://doi.org/10.1172/JCI12131. 103. Hartman ME, Dai DF, Laflamme MA. Human pluripotent stem cells: prospects and challenges as a source of cardiomyocytes for in vitro modeling and cell-based cardiac repair. Adv Drug Deliv Rev. 2016;96:3–17. https://doi.org/10.1016/j.addr.2015.05.004. 107. Lee JH, Lee JB, Shapovalova Z, et al. Somatic transcriptome priming gates lineage-specific differentiation potential of human-induced pluripotent stem cell states. Nat Commun. 2014;5:5605. https://doi.org/10.1038/ncomms6605. 108. Levenberg S, Ferreira LS, Chen-Konak L, Kraehenbuehl TP, Langer R. Isolation, differentiation and characterization of vascular cells derived from human embryonic stem cells. Nat Protoc. 2010;5:1115–26. https://doi.org/10.1038/nprot.2010.31. 111. Kempf H, Olmer R, Kropp C, et al. Controlling expansion and cardiomyogenic differentiation of human pluripotent stem cells in scalable suspension culture. Stem Cell Reports. 2014;3:1132–46. https://doi.org/10.1016/j.stemcr.2014.09.017. 112. Chullikana A, Majumdar AS, Gottipamula S, et al. Randomized, double-blind, phase I/II study of intravenous allogeneic mesenchymal stromal cells in acute myocardial infarction. Cytotherapy. 2015;17:250–61. https://doi.org/10.1016/j.jcyt.2014.10.009. 113. Lee J-W, Lee S-H, Youn Y-J, et al. A randomized, open-label, multicenter trial for the safety and efficacy of adult mesenchymal stem cells after acute myocardial infarction. J Korean Med Sci. 2014;29:23–31. https://doi.org/10.3346/jkms.2014.29.1.23. 114. Bartolucci J, Verdugo FJ, Gonzalez PL, et al. Safety and efficacy of the intravenous infusion of umbilical cord mesenchymal stem cells in patients with heart failure: a phase 1/2 randomized controlled trial (RIMECARD Trial [Randomized Clinical Trial of Intravenous Infusion Umbilical Cord Mesenchymal Stem Cells on Cardiopathy]). Circ Res. 2017;121:1192–204. https://doi.org/10.1161/CIRCRESAHA.117.310712. 115. Kim SH, Cho JH, Lee YH, et al. Improvement in left ventricular function with intracoronary mesenchymal stem cell therapy in a patient with anterior wall ST-segment elevation myocardial infarction. Cardiovasc Drugs Ther. 2018;32:329–38. https://doi.org/10.1007/s10557-018-6804-z. 116. Malliaras K, Makkar RR, Smith RR, et al. Intracoronary cardiosphere-derived cells after myocardial infarction: evidence of therapeutic regeneration in the final 1-year results of the CADUCEUS trial (CArdiosphere-Derived aUtologous stem CElls to reverse ventricUlar dySfunction). J Am Coll Cardiol. 2014;63:110–22. https://doi.org/10.1016/j.jacc.2013.08.724. 117. Bollini S, Silini AR, Banerjee A, Wolbank S, Balbi C, Parolini O. Cardiac restoration stemming from the placenta tree: insights from fetal and perinatal cell biology. Front Physiol. 2018;9:385. https://doi.org/10.3389/fphys.2018.00385. 118. Vadakke-Madathil S, LaRocca G, Raedschelders K, et al. Multipotent fetal-derived Cdx2 cells from placenta regenerate the heart. Proc Natl Acad Sci U S A. 2019;116:11786–95. https://doi.org/10.1073/pnas.1811827116. 119. Hass R, Kasper C, Bohm S, Jacobs R. Different populations and sources of human mesenchymal stem cells (MSC): a comparison of adult and neonatal tissue-derived. MSC Cell Commun Signal. 2011;9:12. https://doi.org/10.1186/1478-811X-9-12. 120. Amable PR, Teixeira MV, Carias RB, Granjeiro JM, Borojevic R. Protein synthesis and secretion in human mesenchymal cells derived from bone marrow, adipose tissue and Wharton’s jelly. Stem Cell Res Ther. 2014;5:53. https://doi.org/10.1186/scrt442. 121. Ayatollahi M, Talaei-Khozani T, Razmkhah M. Growth suppression effect of human mesenchymal stem cells from bone marrow, adipose tissue, and Wharton’s jelly of umbilical cord on PBMCs. Iran J Basic Med Sci. 2016;19:145–53. 122. Karaoz E, Cetinalp Demircan P, Erman G, Gungorurler E, Eker Sariboyaci A. Comparative analyses of immunosuppressive characteristics of bone-marrow, Wharton’s jelly, and adipose tissue-derived human mesenchymal stem cells. Turk J Haematol. 2017;34:213–25. https://doi.org/10.4274/tjh.2016.0171. 123. Ribeiro A, Laranjeira P, Mendes S, et al. Mesenchymal stem cells from umbilical cord matrix, adipose tissue and bone marrow exhibit different capability to suppress peripheral blood B, natural killer and T cells. Stem Cell Res Ther. 2013;4:125. https://doi.org/10.1186/scrt336. 125. Savickiene J, Treigyte G, Baronaite S, et al. Human amniotic fluid mesenchymal stem cells from second- and third-trimester amniocentesis: differentiation potential, molecular signature, and proteome analysis. Stem Cells Int. 2015;2015 319238. https://doi.org/10.1155/2015/319238. 126. Roubelakis MG, Pappa KI, Bitsika V, et al. Molecular and proteomic characterization of human mesenchymal stem cells derived from amniotic fluid: comparison to bone marrow mesenchymal stem cells. Stem Cells Dev. 2007;16:931–52. https://doi.org/10.1089/scd.2007.0036. 127. Pires AO, Mendes-Pinheiro B, Teixeira FG, et al. Unveiling the differences of secretome of human bone marrow mesenchymal stem cells, adipose tissue-derived stem cells, and human umbilical cord perivascular cells: a proteomic analysis. Stem Cells Dev. 2016;25:1073–83. https://doi.org/10.1089/scd.2016.0048. 128. Urbich C, Aicher A, Heeschen C, et al. Soluble factors released by endothelial progenitor cells promote migration of endothelial cells and cardiac resident progenitor cells. J Mol Cell Cardiol. 2005;39:733–42. https://doi.org/10.1016/j.yjmcc.2005.07.003. 129. Adamiak M, Cheng G, Bobis-Wozowicz S, et al. Induced pluripotent stem cell (iPSC)-derived extracellular vesicles are safer and more effective for cardiac repair than iPSCs. Circ Res. 2018;122:296–309. https://doi.org/10.1161/CIRCRESAHA.117.311769. 130. Kervadec A, Bellamy V, El Harane N, et al. Cardiovascular progenitor-derived extracellular vesicles recapitulate the beneficial effects of their parent cells in the treatment of chronic heart failure. J Heart Lung Transplant. 2016;35:795–807. https://doi.org/10.1016/j.healun.2016.01.013. 132. Huang L, Ma W, Ma Y, Feng D, Chen H, Cai B. Exosomes in mesenchymal stem cells, a new therapeutic strategy for cardiovascular diseases? Int J Biol Sci. 2015;11:238–45. https://doi.org/10.7150/ijbs.10725. 133. Arslan F, Lai RC, Smeets MB, et al. Mesenchymal stem cell-derived exosomes increase ATP levels, decrease oxidative stress and activate PI3K/Akt pathway to enhance myocardial viability and prevent adverse remodeling after myocardial ischemia/reperfusion injury. Stem Cell Res. 2013;10:301–12. https://doi.org/10.1016/j.scr.2013.01.002. 135. Barile L, Lionetti V, Cervio E, et al. Extracellular vesicles from human cardiac progenitor cells inhibit cardiomyocyte apoptosis and improve cardiac function after myocardial infarction. Cardiovasc Res. 2014;103:530–41. https://doi.org/10.1093/cvr/cvu167. 136. Zhang M, Methot D, Poppa V, Fujio Y, Walsh K, Murry CE. Cardiomyocyte grafting for cardiac repair: graft cell death and anti-death strategies. J Mol Cell Cardiol. 2001;33:907–21. https://doi.org/10.1006/jmcc.2001.1367. 140. Ban K, Park HJ, Kim S, et al. Cell therapy with embryonic stem cell-derived cardiomyocytes encapsulated in injectable nanomatrix gel enhances cell engraftment and promotes cardiac repair. ACS Nano. 2014;8:10815–25. https://doi.org/10.1021/nn504617g. 141. Chow A, Stuckey DJ, Kidher E, et al. Human induced pluripotent stem cell-derived cardiomyocyte encapsulating bioactive hydrogels improve rat heart function post myocardial infarction. Stem Cell Reports. 2017;9:1415–22. https://doi.org/10.1016/j.stemcr.2017.09.003. 142. Mohanty S, Larsen LB, Trifol J, et al. Fabrication of scalable and structured tissue engineering scaffolds using water dissolvable sacrificial 3D printed moulds. Mater Sci Eng C Mater Biol Appl. 2015;55:569–78. https://doi.org/10.1016/j.msec.2015.06.002. 144. Ribeiro AJ, Ang YS, Fu JD, et al. Contractility of single cardiomyocytes differentiated from pluripotent stem cells depends on physiological shape and substrate stiffness. Proc Natl Acad Sci U S A. 2015;112:12705–10. https://doi.org/10.1073/pnas.1508073112. 145. Gallet R, Dawkins J, Valle J, et al. Exosomes secreted by cardiosphere-derived cells reduce scarring, attenuate adverse remodelling, and improve function in acute and chronic porcine myocardial infarction. Eur Heart J. 2017;38:201–11. https://doi.org/10.1093/eurheartj/ehw240. 146. Shao L, Zhang Y, Lan B, et al. MiRNA-sequence indicates that mesenchymal stem cells and exosomes have similar mechanism to enhance cardiac repair. Biomed Res Int. 2017;2017 4150705. https://doi.org/10.1155/2017/4150705. 147. Tokita Y, Tang XL, Li Q, et al. Repeated administrations of cardiac progenitor cells are markedly more effective than a single administration: a new paradigm in cell therapy. Circ Res. 2016;119:635–51. https://doi.org/10.1161/CIRCRESAHA.116.308937. 148. Florea V, Rieger AC, Natsumeda M, et al. The impact of patient sex on the response to intramyocardial mesenchymal stem cell administration in patients with non-ischemic dilated cardiomyopathy. Cardiovasc Res. 2020 Feb 13; [Epub ahead of print]. https://doi.org/10.1093/cvr/cvaa004. 149. Golpanian S, El-Khorazaty J, Mendizabal A, et al. Effect of aging on human mesenchymal stem cell therapy in ischemic cardiomyopathy patients. J Am Coll Cardiol. 2015;65:125–32. https://doi.org/10.1016/j.jacc.2014.10.040. 150. Luu B, Leistner DM, Herrmann E, et al. Minute myocardial injury as measured by high-sensitive troponin T serum levels predicts the response to intracoronary infusion of bone marrow-derived mononuclear cells in patients with stable chronic post-infarction heart failure: insights from the TOPCARE-CHD registry. Circ Res. 2017;120:1938–46. https://doi.org/10.1161/CIRCRESAHA.116.309938. |