Digestive System
Cyclooxygenase-1 and low levels of COX-2 contribute to gastric mucosal defense, and the isoforms affect different components of this defense.
27,28 Prostaglandins produced by COX-1 regulate the mucosal blood flow and epithelial secretion of mucus and bicarbonate, while PGs produced by COX-2 affect epithelial proliferation and endothelial–leukocyte adherence (
Figure 3).
28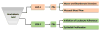 | Figure 3 COX-1 and COX-2 in Gastrointestinal Defense. |
When the COX-2 isoform is inhibited, the absence or underdevelopment of lesions in the stomach indicates that COX-1 is the mediator of gastric mucosal defense under normal conditions.28,29 Surprisingly, it has been reported that gastric lesions do not develop in mice with COX-1 gene knockout; they experience less gastric damage, bleeding, and ulceration than wild-type mice when administered with an NSAID.30 Prostaglandins produced by COX-1 tonically suppress COX-2 activity in the gastrointestinal tract, and the inhibition of COX-1 upregulates COX-2 expression.28,31 In rat intestine, COX-2 expression might increase as a result of the selective inhibition of COX-1. Recent studies have shown that COX-2 plays a role in modulating resistance to lumen irritants when other factors of mucosal defense are genetically or pharmacologically depressed. For example, when nitric oxide (an important mediator of many components of mucosal defense) synthesis is inhibited, selectively inhibiting the COX-2 enzyme leads to stomach damage. Ischemia-reperfusion causes a marked increase in COX-2 expression in the stomach, and the pre-ischemia inhibition of COX-2 was reported to result in further deterioration of the gastric damage. In another study, peritonitis developed in COX-2 knockout mice, possibly due to impairment of the intestinal barrier function.28 In addition, COX-2 null mice are more susceptible to colon damage.29 The COX-2 isoform increases rapidly when mucosal damage occurs or when the COX-1 isoform is inhibited. In these cases, suppressing the activity of the COX-2 isoform results in increased mucosal damage and delayed repair.28
Kidney
Prostaglandins have important physiological roles in the modulation of glomerular hemodynamics, the reabsorption of sodium/water, and the regulation of renin secretion.
15 Both isoforms (but mainly COX-1) are found in the kidney.
8 However, the relevant kidney data are not clear, and the COX-1 and -2 distribution differs according to the organism.
10 In this context, COX-1 is preferentially expressed in the renal vessels and in the papillary and medullary collecting ducts in monkeys, humans, rabbits, dogs, and rats, whereas COX-2 expression is limited in epithelial cells of the thick ascending limb, the macula densa adjacent to the juxtaglomerular apparatus, and medullary interstitial cells of rabbits, rats, and dogs. The expression of COX-2 in adult human kidney occurs in endothelial and smooth muscle cells of the arteries/veins and intra-glomerularly in podocytes. It was not found in macula densa of control kidneys, whereas slight–moderate COX-2 expression was found in the macula densa of patients (46%) with diabetic nephropathy, patients (46%) with hypertension, and patients (30%) with congestive heart failure. Also, COX-2 was determined in patients with Bartter-like syndrome. Additionally, COX-2 expression in adult rat was not detected in the arterioles, glomeruli, and cortical/medullary collecting ducts.
8,32–34
In the kidneys, COX-2 expression in the macula densa depends on the lumenal salt concentration. In rats, COX-2 expression was found to be increased in the macula densa when the salt intake was restricted (at least 3 weeks).32 Cyclooxygenase-2 activates the renin–angiotensin system, while the increased activity of the renin–angiotensin system inhibits the COX-2 isoform.35 Recently, COX-2 was found to be detectable in the macula densa of elderly humans (>60 years of age). It was reported that COX-2 expression in elderly subjects may cause a secondary reduction in basal renin production associated with aging.36 Cyclooxygenase-2 has major importance in healthy volunteers, and rofecoxib, a COX-2 inhibitor, results in blockage of renin and aldosterone secretion.37 Celecoxib is better tolerated in elderly patients, as the risk of acute kidney injury is lower in celecoxib than in rofecoxib.38,39
Although COX enzyme does not play a dominant physiological role in the kidneys of healthy individuals, the COX-1 isoform is primarily involved in the control of renal hemodynamics and the glomerular filtration rate, whereas the COX-2 isoform is primarily involved in salt and water excretion. The COX-2 isoform is regulated in response to intravascular volume. Thus, the inhibition of one or both of these enzymes may have different effects on renal function.9,35
The COX-2-selective inhibitors and NSAIDs may impair the systemic and renal vasodilatory effects of prostacyclin. Loss of this vasodilation effect may lead to increases in systemic vascular resistance and then increases in mean arterial pressure.40 Rofecoxib (not celecoxib or naproxen) caused a significant increase in the systolic blood pressure of osteoarthritis patients.41 Rofecoxib but not celecoxib increased congestive heart failure.42 Non-steroidal anti-inflammatory drugs (COX-2-selective and non-selective) can be used carefully in arthritis patients with stable cardiovascular disorders and hypertension (not including congestive heart failure and moderate–severe kidney dysfunction).40 The COX-2 isoform is expressed in adult and fetal kidney and is required for normal renal development. It has been reported that renal development is impaired in COX-2-deficient mice.43,44 After mother’s use of nimesulide (selective COX-2 inhibitor NSAID) as tocolytic, the lack of effect of nimesulide on fetal renal and ductal function indicates that fetal prostaglandin synthesis is mainly mediated by the COX-1 isoform.45 However, some studies reported neonatal irreversible end-stage renal failure or severe oligohydramnios after use of nimesulide as a tocolytic.46,47
While the COX-1 protein levels did not change in the kidneys of cirrhotic rats, COX-2 protein expression was increased in the corticomedullary region in these animals. Although urinary PGE2 excretion was equally reduced by both selective COX-1 and COX-2 inhibitors in these animals, urinary sodium excretion, the glomerular filtration rate, and renal plasma flow significantly decreased, and the diuretic and natriuretic responses to furosemide were markedly impaired only when COX-1 was selectively inhibited. The selective inhibition of both isoforms in cirrhotic rats did not affect renal water metabolism. These results indicate that preservation of renal function in cirrhotic rats is dependent on the COX-1 isoform despite the abundant expression of renal COX-2 protein.48
Reproductive System
The COX-1 and COX-2 isoforms are present in the reproductive system.
36,49,50 Although both isoforms contribute to the formation of the corpus luteum, COX-1 has been reported to have a greater role than COX-2 in corpus luteum function.
51
Female reproductive functions such as ovulation, implantation, and decidualization are dependent on the COX-2 isoform.36 Multiple disorders of reproductive function relating to ovulation, fertilization, implantation, and decidualization are seen in COX-2-null mice.10,15 The induction of COX-2 is necessary for the successful ruptures of the follicles and likely directly mediates the activation or generation of proteolytic enzymes necessary for ovulation.15 Defective ovulation and failure of fertilization have been reported in female mice lacking the COX-2 isoform. Female mice lacking COX-2 produce fewer eggs, are not fertile, and the development of eggs is abnormal.52 Furthermore, the survival of several offspring born from homozygous COX-1-knockout mice highlights the importance of the COX-1 isoform.30
The COX-1 and COX-2 genes are differentially regulated in the peri-implantation mouse uterus. Cyclooxygenase-1 is involved in decidualization and/or ongoing localized endometrial vascular permeability, while COX-2 is involved in angiogenesis for placenta formation.53 During implantation in humans, the COX-1 isoform is mainly found in the glandular and luminal epithelium, and the COX-2 isoform is primarily found in the luminal epithelium and perivascular cells.54 Initially, COX-2 plays a role in mediating the uterine decidual response, but it is not necessary to sustain embryo development and decidual growth during the remainder of pregnancy.10
Both COX-1 and COX-2 have been identified in the amnion, chorion, and decidua.55 Increased COX-2 expression in the amnion and chorion–decidua was associated with delivery, whereas COX-1 expression was unchanged. In the amnion, COX-1 expression is approximately 100 times lower than COX-2 expression and does not change with delivery.49,56 Expression of COX-2 begins to increase from early pregnancy and reaches its highest level on the 21st day. It was found to be at the lowest level after birth.57 Factors that regulate COX-2 expression in cells and tissues can be specific for physiological processes and for the related tissues. For example, COX-2 expressed in granulosa cells can be induced by luteinizing hormone and follicle-stimulating hormone.3
Balaji et al.58 reported that COX-1 is the dominant isoform in the mouse seminal vesicle. The COX-2 isoform is expressed in the testis, and continuous inhibition of this isoform inhibits sperm maturation.59 The expression levels of COX-1 and COX-2 in sperm are increased under the conditions of varicocele and diabetes.60
Heart and Platelets
The use of NSAIDs and celecoxib or rofecoxib increases risk for cardiovascular events in medical conditions such as diabetes mellitus, congestive heart failure, dyslipidemia, and colorectal adenomas. Additionally, at moderate doses, celecoxib was not found to be inferior to ibuprofen or naproxen in cardiovascular safety.
61–63 Metabolites of COX play important roles in the homeostasis in an organism. The COX-1 isoform is involved in vascular homeostasis in platelets and in most tissues.
14 The only isoform detectable in platelets is COX-1.
9 The role of COX-1 in thromboxane synthesis in platelets is explained by their inability to produce an inducible enzyme in response to activating conditions in seedless platelets.
15
One study demonstrated that prostacyclin in vascular cells is produced by COX-2 and COX-1 under both pathological and physiological conditions.5 Prostacyclin, a potent vasodilator, is one of the most important prostanoids that controls the homeostasis of the cardiovascular system. In addition, it inhibits leukocyte adhesion, platelet aggregation, and the proliferation of vascular smooth muscle cells.4
The function of COX-2 in the cardiovascular system remains largely unknown. Ischemic preconditioning has been shown to increase COX-2 expression and activity in the heart. This increase of COX-2 activity mediates protective effects against myocardial palpitation and myocardial infarction.10
Brain
Although both isoforms have been found in the central nervous system, only the distribution of COX-1 has been reported in detail.
64 The COX-2 isoform is continuously expressed in only a few organs, one of which is the brain.
9 Cyclooxygenase-2 is found in neurons in the cortex and hippocampus of the brain but not in glia or vascular endothelial cells.
65 Medium levels are found in the piriform cortex, pyramidal cells, neocortex, and amygdala. It is found at lower levels in the caudate–putamen, hypothalamus, thalamus, and striatum.
10 The COX-2 isoform, expressed under normal conditions in the central nervous system, contributes to basic brain functions, such as memory consolidation, synaptic activity, and functional hyperemia.
66,67
Cyclooxygenase-2 is highly expressed in the brain of neonates, indicating the importance of this isoform in modulating blood flow at this stage of life.8 Basal expression of COX-2 in developing and adult brain is regulated by natural synaptic activity.65
Bone
Studies have shown that the inhibition or removal of COX-2 inhibits fracture healing, whereas the removal of COX-1 does not affect fracture healing. This indicates that the COX-2 isoform is primarily responsible for regulating fracture healing.
68,69 The healing of tibial fractures was significantly delayed in COX-2
−/− mice compared to COX-1
−/− and wild-type controls.
70 Fracture healing has been reported to be faster with the use of a retroviral vector to express the COX-2 isoform.
68 These results indicate that COX-2 may be a necessary component in fracture healing and that agents modulating COX enzyme activity may directly affect bone repair.
71
Since hemostatic and inflammatory processes are essential components of bone formation, COX enzymes play an important physiological role in fracture healing.71 Fracture healing is a complex three-stage process: (1) inflammation stage, (2) reparative stage, and (3) remodeling stage. The first stage begins immediately after fracture and is characterized by the formation of a hematoma, the migration of mesenchymal cells to the fracture site, and the release of growth factors and cytokines from fibroblasts and leukocytes. Following the initial inflammatory response, new bone is formed by intramembranous and endochondral ossification; these processes are mostly mediated by osteoblasts. These stages are followed by the long process of remodeling. Fracture healing is similar to many aspects of bone growth, but COX-2-mediated inflammation is an important initiation step in the fracture-healing process.10,72
Inflammation
The COX-2 isoform is reported to play a central role in the inflammation process, and inhibition of this isoform is sufficient to achieve the same therapeutic results as less specific inhibitors that also target the COX-1 isoform (
Figure 4).
15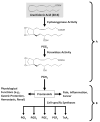 | Figure 4 Biosynthesis and Main Biological Activities of Prostanoid Mediators from Arachidonic Acid. |
Although the role of COX-2 is not fully understood, the relationship between its induction and nerve degeneration suggests that the COX-2 isoform may play a greater role in the selective loss of nerve connections.8 Central inhibition of the COX-2 isoform may be important in pain control.10 Prostaglandin E2 (PGE2) synthesis is induced by cytokines, which are released by the action of pyrogens such as lipopolysaccharide. Although COX-2 expression in the central nervous system is increased after lipopolysaccharide stimulation, the induction occurs in the endothelium of cranial blood vessels and in the microglia, not in neurons. Furthermore, it is clear that PGE2 in the febrile response is caused by the COX-2 isoform induced in endothelial cells of blood vessels perfusing hypothalamus in non-neuronal cells.9
In epithelial cells, COX-2 expression increases during inflammation.15 An increase in COX-2 levels as a result of an intrauterine infection was found to be associated with preterm delivery.73 The expression of COX-2 during wound healing contributes to the wound healing process. In animal wound models, COX-2 inhibition was shown to exacerbate inflammation and to delay wound healing.15
In joint inflammation models, COX-2 expression was increased but COX-1 expression remained unchanged.74,75 The role of COX-1 and COX-2 in chronic pain such as rheumatoid and osteoarthritis continues to be investigated.10
Parkinson’s and Alzheimer’s Disease
The overexpression of COX-2 has been reported in neurodegenerative disorders.
26 The COX-2 isoform increases brain parenchymal amyloid plaque formation, leading to Alzheimer’s disease. Relatedly, the inhibition of this isoform has been reported to reduce the risk of Alzheimer’s disease.
8 However, Aisen et al.
76 reported that low-dose naproxen or rofecoxib did not slow cognitive decline in mild–moderate Alzheimer’s disease.
Cancer
Many studies have reported that COX enzymes play an important role in the development of tumors by affecting angiogenesis in tumor cells.
5,15 The roles of the two isoforms in tumorigenesis are different. The COX-1 isoform is expressed in vascular endothelial cells and contributes to angiogenesis that is involved in tumor or endometrial growth, wound healing, and inflammation. Unlike the relatively small contribution of the COX-1 isoform to tumorigenesis, COX-2 is functional in tumor formation and tumor growth. The overexpression of COX-2 in tumor cells causes the cells to escape from apoptosis and invade the matrix.
5
Many studies have shown that COX-2 is overexpressed in some pre-cancerous lesions and cancer types, including kidney, uterus, bowel, and bladder cancer.14,36,77 The contribution of COX-2 to the development of colon cancers supports a role in the control of cell growth that is apart from the COX-1 isoform.3 In human gastric adenocarcinoma tissues, COX-2 mRNA levels were increased compared to normal samples in gastric mucosal tissue, but COX-1 mRNA levels were not elevated in carcinoma.9 The prolonged increase of COX-2 expression following colitis increases the sensitivity to colon cancer.28